Xylella fastidiosa(XYLEFA)
EPPO Datasheet: Xylella fastidiosa
IDENTITY
Authority: Wells, Raju, Hung, Weisburg, Parl & Beemer
Taxonomic position: Bacteria: Proteobacteria: Gammaproteobacteria: Lysobacterales: Lysobacteraceae
Other scientific names: Grapevine Pierce's disease agent, Xylella fastidiosa subsp. piercei Schaad et al.
Common names in English: Anaheim disease, California vine disease, Pierce's disease of grapevine, citrus variegated chlorosis, dwarf disease of alfalfa (US), dwarf disease of lucerne (GB), leaf scorch of American sycamore, leaf scorch of almond, leaf scorch of coffee, leaf scorch of elm, leaf scorch of maple, leaf scorch of mulberry, leaf scorch of oleander, olive quick decline syndrome, peach phony disease, plum leaf scald
view more common names online...
Notes on taxonomy and nomenclature
X. fastidiosa is a genetically diverse species that has been associated with a wide range of plant diseases (see common names above).
Subspecies: six different subspecies of X. fastidiosa have been proposed, namely:
- X. fastidiosa subsp. fastidiosa
- X. fastidiosa subsp. multiplex
- X. fastidiosa subsp. pauca
- X. fastidiosa subsp. sandyi
- X. fastidiosa subsp. tashke
- X. fastidiosa subsp. morus
However, of these subspecies only two, fastidiosa and multiplex, are currently officially considered as valid names by the International Society of Plant Pathology Committee on the Taxonomy of Plant Pathogenic Bacteria (ISPP-CTPPB) (Bull et al., 2012). A pathovar subdivision within X. fastidiosa has also been proposed (Hendson et al., 2001).
Sequence types (STs): Multilocus sequence typing (Maiden et al., 1998) is a genetic typing methodology that is widely used to characterise X. fastidiosa as well as other bacteria (Scally et al., 2005; Yuan et al., 2010; Nunney et al., 2014; Denancé et al., 2017, 2019). Different STs have been identified in the different outbreaks in the EPPO region: ST53 (X. fastidiosa subsp. pauca) and ST87 (X. fastidiosa subsp. multiplex) in Italy; ST6 (X. fastidiosa subsp. multiplex), ST7 (X. fastidiosa subsp. multiplex) and ST53 in France; ST7 in Portugal; ST1 (X. fastidiosa subsp. fastidiosa), ST6, ST7, ST80 (X. fastidiosa subsp. pauca), and ST81 (X. fastidiosa subsp. multiplex) in Spain (Balearic Islands).
EU Categorization: Emergency measures, A2 Quarantine pest (Annex II B)
view more categorizations online...
EPPO Code: XYLEFA
HOSTS 2020-05-05
X. fastidiosa is a polyphagous bacterium that can infect the xylem of a wide range of cultivated and wild host plants. A total of 595 plant species have been reported in the scientific literature (EFSA, 2020) as plant hosts of X. fastidiosa. From these, 343 were confirmed using at least two different molecular tests for the detection of the bacterium, and many of these plant species were found to have been infected in natural conditions.
X. fastidiosa is known to cause severe direct damage to several major crops including grapevine, almond, coffee, citrus, stone fruits, as well as forest, landscape and ornamental trees. The main and most common diseases caused by the bacterium are Pierce’s disease (PD) of grapevine in North America, citrus variegated chlorosis (CVC) in South America, and, in recent years, olive quick decline syndrome (OQDS).
The OQDS is a devasting disease caused by X. fastidiosa that emerged in Southern Italy in 2013 (Saponari et al., 2013) but that was first detected in the USA in the 2000s (Hernandez-Martinez, 2007; Krugner, 2011). The disease on olive (also called olive leaf scorch and quick decline) has also emerged in Argentina and Brazil (Haelterman et al., 2015; Coletta-Filho et al., 2016).
In the European Union territory, a list of host plants found to be susceptible to Xylella fastidiosa and its subspecies is maintained in a database (European Commission, 2020). It can be noted that no single strain of X. fastidiosa can infect all of the plant hosts and a large host range variation has been ascertained even within subspecies and among strains sharing the same ST (Nunney et al., 2019).
Host list: Acacia cultriformis, Acacia dealbata, Acacia longifolia, Acacia melanoxylon, Acacia saligna, Acacia sp., Acer granatense, Acer griseum, Acer macrophyllum, Acer negundo, Acer platanoides, Acer pseudoplatanus, Acer rubrum, Acer saccharum, Acer sp., Adenocarpus lainzii, Adenocarpus sp., Aesculus x hybrida, Agathis australis, Agrostis gigantea, Ailanthus altissima, Albizia julibrissin, Alectryon excelsus, Alnus rhombifolia, Alternanthera ficoidea, Amaranthus retroflexus, Amaranthus sp., Ambrosia artemisiifolia, Ambrosia psilostachya, Ambrosia sp., Ambrosia trifida var. texana, Ambrosia trifida, Ampelopsis arborea, Ampelopsis brevipedunculata var. hancei, Ampelopsis cordata, Ampelopsis glandulosa var. brevipedunculata, Anthyllis barba-jovis, Anthyllis hermanniae, Arbutus unedo, Arctostaphylos sp., Argyranthemum frutescens, Artemisia absinthium, Artemisia arborescens, Artemisia douglasiana, Artemisia sp., Asparagus acutifolius, Athyrium filix-femina, Atriplex sp., Avena fatua, Axonopus compressus, Baccharis halimifolia, Baccharis pilularis, Baccharis sp., Berberis thunbergii, Bidens pilosa, Boerhavia diffusa, Brachiaria plantaginea, Brachyglottis compacta, Brachyglottis sp., Brassica sp., Bromus diandrus, Bromus rigidus, Bromus sp., Broussonetia papyrifera, Calicotome sp., Calicotome spinosa, Calicotome villosa, Callicarpa americana, Calluna vulgaris, Calyptocarpus biaristatus, Campsis radicans, Capsella bursa-pastoris, Carex sp., Carpinus caroliniana, Carya aquatica, Carya cathayensis, Carya cordiformis, Carya floridana, Carya glabra, Carya illinoinensis, Carya laciniosa, Carya pallida, Carya sp., Carya tomentosa, Castanea sativa, Catharanthus roseus, Catharanthus, Celastrus orbiculatus, Celtis occidentalis, Celtis sp., Cenchrus clandestinus, Cenchrus echinatus, Cercis canadensis, Cercis occidentalis, Cercis siliquastrum, Chamaecrista fasciculata, Chenopodiastrum murale, Chenopodium album, Chionanthus retusus, Chionanthus sp., Chloris halophila, Cistus albidus, Cistus creticus, Cistus inflatus, Cistus ladanifer, Cistus monspeliensis, Cistus salviifolius, Cistus sp., Cistus x incanus, Citrus celebica, Citrus medica, Citrus natsudaidai, Citrus reticulata, Citrus sp., Citrus x aurantium var. paradisi, Citrus x aurantium var. sinensis, Citrus x aurantium var. tangerina, Citrus x aurantium, Citrus x limon, Citrus x limonia var. jambhiri, Citrus x limonia, Citrus x nobilis, Citrus x tangelo, Clematis cirrhosa, Clematis vitalba, Clianthus puniceus, Clinopodium nepeta, Coelorachis cylindrica, Coffea arabica, Coffea canephora, Coffea eugenioides, Coffea excelsa, Coffea hybrids, Coffea kapakata, Coffea liberica, Coffea sp., Coffea stenophylla, Coleonema album, Commelina benghalensis, Commelina erecta, Conium maculatum, Convolvulus arvensis, Convolvulus cneorum, Coprosma repens, Coprosma robusta, Cordyline australis, Cordyline sp., Cornus florida, Cornus sanguinea, Corokia cotoneaster, Corokia macrocarpa, Corokia sp., Coronilla valentina subsp. glauca, Coronilla valentina, Cortaderia selloana, Corynocarpus laevigatus, Croton setigerus, Cynodon dactylon, Cyperus eragrostis, Cyperus sp., Cytisus multiflorus, Cytisus scoparius, Cytisus sp., Cytisus striatus, Cytisus villosus, Datura wrightii, Dichanthelium acuminatum, Digitaria horizontalis, Digitaria insularis, Digitaria sanguinalis, Digitaria sp., Dimorphotheca ecklonis, Dimorphotheca fruticosa, Diospyros kaki, Diplocyclos palmatus, Distimake macrocalyx, Dittrichia viscosa, Dodonaea viscosa, Duranta erecta, Dysphania ambrosioides, Echinochloa crus-galli, Echinospartum lusitanicum, Echium plantagineum, Elaeagnus angustifolia, Elaeagnus x submacrophylla, Eleusine indica, Encelia farinosa, Eremophila maculata, Erica cinerea, Erigeron bonariensis, Erigeron canadensis, Erigeron karvinskianus, Erigeron sp., Erigeron sumatrensis, Eriocephalus africanus, Eriochloa contracta, Eriogonum sp., Erodium botrys, Erodium moschatum, Erodium sp., Erysimum hybrids, Erysimum, Escallonia bifida, Eucalyptus sp., Euphorbia chamaesyce, Euphorbia hirta, Euphorbia terracina, Euryops chrysanthemoides, Euryops pectinatus, Facelis retusa, Fagus crenata, Fatsia japonica, Ficus carica, Fragaria vesca subsp. bracteata, Frangula alnus, Fraxinus americana, Fraxinus angustifolia, Fraxinus dipetala, Fraxinus excelsior, Fraxinus pennsylvanica, Fraxinus sp., Fuchsia magellanica, Gahnia sp., Gazania rigens, Genista balearica, Genista corsica, Genista ephedroides, Genista hirsuta, Genista scorpius, Genista sp., Genista triacanthos, Genista tricuspidata, Genista tridentata, Genista x spachiana, Geranium dissectum, Ginkgo biloba, Gleditsia triacanthos, Grevillea juniperina, Grevillea rosmarinifolia, Halimium calycinum, Halimium lasianthum, Halimium ocymoides, Halimium sp., Haloragis erecta, Hedera helix, Helianthus annuus, Helianthus sp., Helichrysum italicum, Helichrysum sp., Helichrysum stoechas, Heliotropium europaeum, Heliotropium fruticosum, Heliotropium indicum, Hemerocallis sp., Heterotheca grandiflora, Hevea brasiliensis, Hibiscus rosa-sinensis, Hibiscus schizopetalus, Hibiscus sp., Hibiscus syriacus, Hordeum murinum, Humulus scandens, Hydrangea paniculata, Hypericum androsaemum, Hypericum perforatum, Hypochaeris brasiliensis, Ilex aquifolium, Ilex vomitoria, Ipomoea carnea subsp. fistulosa, Iva annua, Jacaranda mimosifolia, Jacobaea maritima, Juglans regia, Juniperus ashei, Koelreuteria bipinnata, Lactuca serriola, Lagerstroemia indica, Lagerstroemia sp., Laurus nobilis, Laurus sp., Lavandula angustifolia, Lavandula dentata, Lavandula latifolia, Lavandula sp., Lavandula stoechas, Lavandula x chaytorae, Lavandula x heterophylla, Lavandula x intermedia, Leonurus sibiricus, Lepidium auriculatum, Lepidium didymum, Lepidium ruderale, Leucophyta brownii, Libertia peregrinans, Ligustrum lucidum, Ligustrum sinense, Liquidambar styraciflua, Liriodendron tulipifera, Lolium multiflorum, Lolium perenne, Lonicera implexa, Lonicera japonica, Lonicera periclymenum, Lonicera sp., Ludwigia grandiflora, Lupinus aridorum, Lupinus villosus, Magnolia grandiflora, Magnolia x soulangeana, Mallotus paniculatus, Malva multiflora, Malva parviflora, Marrubium vulgare, Medicago arborea, Medicago polymorpha, Medicago sativa, Melaleuca citrina, Melicope ternata, Melicytus ramiflorus, Melilotus sp., Melissa officinalis, Mentha suaveolens, Meryta sinclairii, Metrosideros excelsa, Metrosideros kermadecensis, Metrosideros sp., Mimosa sp., Modiola caroliniana, Montia linearis, Morus alba, Morus rubra, Morus sp., Myoporum insulare, Myoporum laetum, Myoporum sp., Myrtus communis, Nandina domestica, Neptunia lutea, Nerium oleander, Olea europaea subsp. sylvestris, Olea europaea, Olea sp., Olearia traversii, Origanum majorana, Pachystegia insignis, Parthenium hysterophorus, Parthenocissus quinquefolia, Parthenocissus tricuspidata, Paspalum dilatatum, Paspalum regnellii, Paspalum urvillei, Passiflora foetida, Pelargonium graveolens, Pelargonium sp., Pelargonium x fragrans, Persea americana, Persicaria lapathifolia, Persicaria maculosa, Phagnalon saxatile, Phagnalon sp., Phalaris angusta, Phillyrea angustifolia, Phillyrea latifolia, Phlomis fruticosa, Phlomis italica, Phoenix reclinata, Phoenix roebelenii, Phoenix sp., Phormium colensoi, Phormium tenax, Phyllocladus trichomanoides var. alpinus, Pinus taeda, Pistacia vera, Pittosporum crassifolium, Pittosporum eugenioides, Pittosporum tenuifolium, Pittosporum umbellatum, Plantago lanceolata, Plantago major, Platanus occidentalis, Platanus sp., Platanus x hispanica, Pluchea odorata, Poa annua, Polygala myrtifolia, Polygala sp., Polygala x dalmaisiana, Polygonum arenastrum, Portulaca oleracea, Prunus americana, Prunus angustifolia, Prunus armeniaca, Prunus avium, Prunus campanulata, Prunus cerasifera, Prunus cerasus, Prunus domestica, Prunus dulcis, Prunus hortulana, Prunus hybrids, Prunus laurocerasus, Prunus mexicana, Prunus mume, Prunus munsoniana, Prunus persica, Prunus salicina, Prunus serotina, Prunus serrulata, Prunus simonii, Prunus sp., Psidium sp., Pteridium aquilinum, Pyracantha coccinea, Pyrus pyrifolia, Pyrus sp., Quercus agrifolia, Quercus alba, Quercus cerris, Quercus coccinea, Quercus falcata, Quercus ilex, Quercus imbricaria, Quercus incana, Quercus laevis, Quercus laurifolia, Quercus macrocarpa, Quercus nigra, Quercus palustris, Quercus phellos, Quercus prinus, Quercus pubescens, Quercus pyrenaica, Quercus robur, Quercus rubra, Quercus shumardii, Quercus sp., Quercus suber, Quercus velutina, Quercus virginiana, Ranunculus repens, Raphanus sativus, Ratibida columnifera, Retama monosperma, Reynoutria japonica, Rhamnus alaternus, Rhamnus sp., Rhus sp., Richardia sp., Robinia pseudoacacia, Rosa californica, Rosa canina, Rosa sp., Rubus idaeus, Rubus procerus, Rubus rigidus, Rubus sp., Rubus ulmifolius, Rubus ursinus, Rubus vitifolius, Rudgea verticillata, Rumex crispus, Rumex sp., Ruta chalepensis, Ruta graveolens, Salix atrocinerea, Salix sp., Salsola tragus, Salvia abrotanoides, Salvia mellifera, Salvia officinalis, Salvia rosmarinus, Salvia sp., Sambucus canadensis, Sambucus cerulea, Sambucus nigra, Sambucus sp., Santolina chamaecyparissus, Santolina magonica, Santolina sp., Sapindus saponaria, Sassafras albidum, Sassafras sp., Scabiosa atropurpurea var. maritima, Senecio grisebachii, Senecio inaequidens, Senecio vulgaris, Setaria magna, Sida rhombifolia, Silybum marianum, Sisymbrium irio, Solanum americanum, Solidago canadensis, Solidago fistulosa, Solidago virgaurea, Sonchus oleraceus, Sonchus sp., Sophora secundiflora, Sorghum halepense, Spartium junceum, Spartium sp., Spermacoce latifolia, Stachys arvensis, Stellaria media, Stewartia pseudocamellia, Strelitzia reginae, Streptocarpus hybrids, Streptocarpus, Symphyotrichum divaricatum, Syringa vulgaris, Syzygium paniculatum, Talinum paniculatum, Taraxacum officinale, Teucrium capitatum, Thymus vulgaris, Toxicodendron diversilobum, Trifolium incarnatum, Trifolium repens, Ulex europaeus, Ulex micranthus, Ulex minor, Ulex parviflorus, Ulex sp., Ulmus americana, Ulmus crassifolia, Ulmus glabra, Ulmus pumila, Ulmus sp., Ulmus x hollandica, Urochloa eminii, Urtica dioica subsp. gracilis, Urtica urens, Vaccinium ashei, Vaccinium corymbosum, Vaccinium darrowii, Vaccinium sp., Vaccinium virgatum, Verbena litoralis, Vernonia sp., Veronica elliptica, Veronica persica, Veronica sp., Veronica, Viburnum tinus, Vicia ludoviciana, Vinca major, Vinca minor, Vinca sp., Vinca, Vitex agnus-castus, Vitex lucens, Vitis aestivalis, Vitis arizonica, Vitis bourquiniana, Vitis californica, Vitis candicans, Vitis cinerea var. floridana, Vitis cinerea, Vitis girdiana, Vitis hybrids, Vitis labrusca, Vitis munsoniana, Vitis riparia, Vitis rotundifolia, Vitis shuttleworthii, Vitis sp., Vitis vinifera, Vitis x champinii, Westringia fruticosa, Westringia glabra, Wisteria frutescens, Xanthium spinosum, Xanthium strumarium, x Chitalpa tashkentensisGEOGRAPHICAL DISTRIBUTION 2020-05-05
X. fastidiosa is known to occur over a wide range of climatic zones in tropical and subtropical areas, as well as in more temperate or even continental climate regions. Until the 2010s, X. fastidiosa was known to occur in the Americas only. In Asia, a bacterium causing pear leaf scorch disease in Taiwan and first identified as X. fastidiosa (Su et al., 2013) has later been reported as being a new species X. taiwanensis (Su et al., 2016). In the EPPO region, the outbreak of X. fastidiosa in olive trees in Apulia, Southern Italy (Saponari et al., 2013) and the presence of the bacterium in Mediterranean plant species in the natural and urban landscapes of Italy (Apulia, Toscana), France (Corsica and Provence-Alpes-Côte d’Azur), Spain (Balearic Islands, Comunidad Valenciana and Madrid) and Portugal (Porto), constituted a major change to its geographical distribution. Records from Turkey (Güldür et al., 2005), Lebanon (Temsah et al., 2015; Habib et al., 2016) and Kosovo (Berisha et al., 1998; EPPO, 1998) are considered invalid.
The distribution below is given for all X. fastidiosa subspecies.
Asia: China (Xinjiang), Iran, Islamic Republic of, Iraq, Israel, Lebanon, Taiwan
North America: Canada (British Columbia, Ontario, Saskatchewan), Mexico, United States of America (Alabama, Arizona, Arkansas, California, Delaware, District of Columbia, Florida, Georgia, Indiana, Kentucky, Louisiana, Maryland, Mississippi, Missouri, New Jersey, New Mexico, New York, North Carolina, Oklahoma, Oregon, Pennsylvania, Rhode Island, South Carolina, Tennessee, Texas, Virginia, Washington, West Virginia)
Central America and Caribbean: Costa Rica, Puerto Rico
South America: Argentina, Brazil (Alagoas, Amazonas, Bahia, Distrito Federal, Espirito Santo, Goias, Minas Gerais, Para, Parana, Rio de Janeiro, Rio Grande do Sul, Santa Catarina, Sao Paulo, Sergipe), Colombia, Paraguay, Peru, Venezuela
BIOLOGY 2020-05-05
Interaction between X. fastidiosa and its host plants
Xylella fastidiosa is a xylem-limited fastidious bacterium that in nature is exclusively transmitted by xylem-fluid feeding insects. The bacterium therefore proliferates only in xylem vessels, in roots, stems and leaves. Symptom expression is usually linked to the occlusion of these vessels by bacterial aggregates, as well as by tyloses and gums formed by the plant. Therefore, symptoms of X. fastidiosa often resemble those caused by water stress. In some cases, the infection results in rapid death of the host plant. However, many plant species if not the majority may not even express any symptoms (EFSA, 2015a, 2018, 2019b). The symptomless plants often serve as a source of inoculum for vectors (Hopkins and Purcell, 2002). In some host plants, bacterial cells may remain restricted to parts of the plant or even at the point of entrance. In susceptible plants, they move systemically through the xylem vessels, mainly upstream but also downstream, and may invade all plant organs. The time period between infection and the appearance of symptoms (incubation period) is highly variable and ranges from a few months to even more than one year, depending on the X. fastidiosa genotype, the host species, the age of the plant and growing conditions (EFSA, 2019b, 2020).
Winter climate is usually a key factor in delimiting the areas where X. fastidiosa can persist from one season to the next. Pierce's disease and phony disease only occur in areas with a mild winter, presumably in relation to survival of the bacterium in dormant plants (Purcell, 1989). Experimental cold therapy of diseased grapevines suggests that freezing temperatures can eliminate the bacterium directly in the plants (Purcell, 1980). Although the bacterium preferably overwinters in infected plants, it may also survive in its insect vectors. X. fastidiosa is efficiently acquired by vector insects, with no latent period, and it multiplies and persists in infective adults indefinitely (Severin, 1950). Therefore, if these infected adults overwinter, they can maintain X. fastidiosa during the adverse season. This is the case of sharpshooters (Cicadellidae, Cicadellinae) in the American continent. However, the European sharpshooters and most of the European spittlebugs (Aphrophoridae and a few Cercopidae) overwinter as eggs and therefore, if infected, these vectors cannot sustain overwintering of X. fastidiosa, since transovarial transmission of X. fastidiosa does not occur (Freitag, 1951).
Interaction between X. fastidiosa and its vectors
In theory all xylem-fluid feeding insects are considered vectors, until proven otherwise (Frazier, 1944; Purcell, 1989; Almeida et al., 2005), but transmission efficiency varies substantially depending on insect species, host plant, and X. fastidiosa genotype (Redak et al. 2004; Lopes et al., 2010). All X. fastidiosa insect vectors belong to Auchenorrhyncha (Hemiptera) and are distributed within the superfamilies Cercopoidea, Cicadoidea and Membracoidea. Each of the three superfamilies include xylem-fluid feeding groups, but, while all Cercopoidea (known as spittlebugs or froghoppers) and Cicadoidea (cicadas) are regarded as xylem-fluid feeders, in Membracoidea only the subfamily Cicadellinae (known as sharpshooters) within the family Cicadellidae are actually xylem-fluid feeders.
Acquired bacteria adhere to the precibarium and the cibarium of the foregut and do not systemically colonize the insect body (Purcell and Finlay, 1979). This implies that nymphs lose infectivity when moulting, as the foregut is of ectodermal origin and is renewed, and that newly emerged adults must again acquire X. fastidiosa to become infectious. Normally very few live bacterial cells are sufficient for successful transmission (Hill and Purcell, 1995, 1997) which is neither transovarial nor transstadial. Temperature has a key role in the interaction between the bacterium and its vectors: it influences the pathogen multiplication in source plants (Feil and Purcell, 2001); the pathogen multiplication in vectors (Dohm et al., 2002); the successful establishment of the pathogen in the new host (Chu and Volety, 1997), and the vector behaviour (Su and Mulla, 2001).
In Californian vineyards, Homalodisca vitripennis (=H. coagulata), Xyphon fulgidum (=Carneocephala fulgida), Draeculacephala minerva, and Graphocephala atropunctata are considered to be the most important vectors of Pierce's disease. In Brazilian citrus orchards, Acrogonia terminalis, Dilobopterus costalimai, Oncometopia facialis are considered to be the most important vectors of citrus variegated chlorosis.
In the EPPO region, the only confirmed vectors of X. fastidiosa are Philaenus spumarius (Cercopoidea: Aphrophoridae), and in experimental conditions, Neophilaenus campestris and Philaenus italosignus (Aphrophoridae) (Cavalieri et al., 2018; Saponari et al., 2014, Cornara et al., 2017b). P. spumarius (meadow spittlebug), the only effective vector of X. fastidiosa under natural conditions, is a highly polyphagous and univoltine species. Nymphs and adults have a different host range. The nymphs prefer tender plant parts and herbaceous plants, in particular plants of the Asteraceae, Fabaceae, and Apiaceae families (Di Serio et al., 2019; Dongiovanni et al., 2018b) which often grow as cover crops or invasive plants, in the olive groves, on field hedges, and in natural and semi-natural areas. The adults, by contrast, tend to feed on woody plant species (EFSA, 2019c).
In Southern Italy (Apulia) adults of P. spumarius emerge between the end of April and the beginning of May and move from the herbaceous vegetation of olive groves to the canopy of olive trees and other evergreen or deciduous trees and shrubs in late spring to early summer (Di Serio et al., 2019; Cornara et al., 2017a; Bodino et al., 2017). This movement can also be observed if the grass cover persists over the summer. At the end of summer and early autumn, adults return to herbaceous plants for egg laying and overwintering (Weaver and King, 1954; Cornara et al., 2018). The host range of X. fastidiosa subsp. pauca strain ST53, which causes the OQDS in Italy, overlaps with that of P. spumarius (Weaver and King, 1954; Cornara et al., 2017b), suggesting that some of these host species may serve as natural reservoirs and possibly as inoculum sources for primary spread of the pathogen to the affected crops.
Climatic conditions in different parts of the EPPO region can affect the life cycle of P. spumarius. For example, in the Mediterranean climate, adults are abundant soon after emergence and then later after aestivation in October. In the Netherlands, by contrast, P. spumarius can be detected from June onwards to October (Noordijk et al., 2019), with a peak in the adult population in June and July.
In general, in the context of surveillance and considering the above, it is preferable to collect insect samples late in the season, as insects collected during this period might have fed on multiple host plants and the chances to be infected are therefore higher.
DETECTION AND IDENTIFICATION 2023-09-07
The detection and identification of Xylella fastidiosa relies on the combination of visual examination, sampling and testing of insect vectors and plant material.
Symptoms
Symptoms of Xylella-diseases are highly variable and their expression depends on the combination of host plant and bacterial strain, as well as on environmental conditions, including the specific growing conditions of the individual plant and its phenological stage. These symptoms include leaf scorching, wilting of the foliage, (premature) defoliation, chlorosis or bronzing along the leaf margin, stunting and dieback of shoots and twigs, formation of new malformed (asymmetric) leaves and dwarfing (EPPO, 2023). Considering the large host range of X. fastidiosa, descriptions of symptoms are provided below only for its main hosts.
Grapevine
The most characteristic symptom of primary infection is leaf scorch. An early sign is the sudden drying of part of a green leaf, which then turns brown while adjacent tissues turn yellow or red. The desiccation spreads and the whole leaf may shrivel and drop, leaving only the petiole attached. Diseased stems often mature irregularly, with patches of brown and green tissue. In later years, infected plants develop late and produce stunted chlorotic shoots. They rarely survive more than a year or two, even if there are signs of recovery.
Peach
Young shoots are stunted and bear greener, denser foliage than healthy trees. Lateral branches grow horizontally or droop, so that the tree seems uniform, compact and rounded. Leaves and flowers appear early, and leaves remain on the tree longer than on healthy trees. Affected trees yield increasingly fewer and smaller fruits until, after 3-5 years, they become economically worthless.
Citrus
Trees can start showing the symptoms of variegated chlorosis from nursery size up to 7-10 years of age. These younger trees become systemically affected by X. fastidiosa. Trees more than 15 years old are not usually totally affected, but rather have one or two major scaffold branches showing symptoms. Affected trees show foliar chlorosis resembling zinc deficiency with interveinal chlorosis. The chlorosis appears on young leaves as they mature and may also occur on older leaves. Newly affected trees show sectoring of symptoms, whereas trees which have been affected for a period of time show the variegated chlorosis throughout the canopy. As the leaves mature, small, light-brown, slightly raised gummy lesions (becoming dark-brown or even necrotic) appear on the underside, corresponding to the yellow chlorotic areas on the upper side.
Fruit size is greatly reduced; its sugar content is higher than in non-affected fruit, and the rind may become so hard that can cause damage to juicing machines. Blossom and fruit set occur at the same time on healthy and affected trees, but physiological fruit thinning does not occur on affected trees and the fruits remain small. Because of this, total production is not greatly reduced. On affected trees of cv. Pera and other orange cultivars, fruits often occur in clusters of 4-10, resembling grape clusters. Affected trees show stunting and slow growth rate; twigs and branches die back, although usually the plant does not die, and the canopy thins.
Olive
The disease on olive plants is characterized by the appearance of scattered desiccation of twigs and small branches. Leaves are the first to be affected. Scorching starts at their tip and progresses towards the petiole, extending to the whole blade. Dead leaves remain attached throughout summer to the twigs, which are also desiccating, and begin to drop with the first rains in autumn. Symptoms are first localized in the upper part of the crown, then they extend to the rest of the canopy. Trees of susceptible cultivars, decline and die within a few years from the appearance of symptoms. These trees, especially the centuries-old ones, are often pruned heavily, forcing them to push new growth which, eventually, will wither and desiccate (Martelli, 2016).
Polygala myrtifolia
This is one of the major susceptible hosts in the current European outbreak. Infected plants show scorched leaves, with desiccation starting from the tip and progressing to the entire blade.
Morphology
Xylella fastidiosa
X. fastidiosa is a Gram-negative bacterium, rod-shaped with distinctive rippled cell walls. It is non-flagellate, does not form spores and measures 0.1-0.5 x 1-5 µm.
Vectors
Adult sharpshooters are distinguished from other leafhopper subfamilies by the possession of highly ‘inflated’ or ‘swollen’ faces caused by the massive musculature required by the cibarial (pharyngeal) pump to suck in large quantities of xylem (Young, 1968). Sharpshooters are typically among the largest of leafhoppers. The smaller species (e.g. Xyphon fulgidum) may be about 4 mm in length, while larger species such as Homalodisca vitripennis may exceed 15 mm. Nymphs generally resemble adults in form but usually differ markedly in colouration and markings. The subfamily is quite diverse, especially in the American tropics, with over 210 genera (Young, 1977). Colouration varies from uniformly green (e.g. Draeculacephala spp.) or other cryptic colouration to combinations of bright-red, orange, yellow and blue tropical species. Sharpshooters typically have broad plant host ranges. Even those species that are found on a single or few host species are capable of prolonged survival in captivity on unusual hosts. The rhododendron sharpshooter, Graphocephala fennahi, is a North American species now established in Europe, where it occurs not only on Rhododendron but on many other woody plants. Female sharpshooters and spittlebugs insert their eggs into plant tissues. The eggs of some sharpshooter species remain dormant over winter.
Cercopidae may be distinguished from leafhoppers, which possess one or more rows of spines along the hind tibia, by the smooth hind tibia with a few or a circlet of stout spines at the apex of the tibia. The body shape of froghoppers is generally stouter than that of leafhoppers.
The meadow spittlebug Philaenus spumarius belongs to the order Hemiptera, superfamily Cercopoidea, family Aphrophoridae. The name spittlebug came from the shell built up by the nymphs mixing fluid voided from the anus and a secretion produced by glands located between the 7th and the 8th abdominal sternites. Air bubbles are introduced within the spittle by mean of caudal appendages and a ventral tube formed by abdominal tergites bent downward (Cornara et al., 2018).
P. spumarius, that has never been considered an agricultural pest in Europe before the introduction of X. fastidiosa, is extremely variable in colour, ranging from light grey to blackish although the most typical form is yellow-green with indistinct dark lines.
P. spumarius is widely distributed (temperate regions of Europe, Asia, North America and also North Africa) and occurs in most terrestrial habitats (meadows, abandoned fields, waste ground, roadsides, banks of streams, hayfields, marshlands, parks, gardens and cultivated fields; Yurtsever, 2000) on hundreds of host plants ranging from grasses to trees, including meadow crops, herbs, thistles, garden plants, shrubs, and conifers. However, nitrogen fixing legumes and other plants with high amino acids concentration in the xylem sap (Medicago sativa, Trifolium sp., Vicia spp., and Xanthium strumarium) are the preferred hosts.
P. spumarius overwinter as eggs. Eggs are oviposited in stubble, herbs, dead parts of plants, plant residues, cracks and tree trunk barks, or in the leaf litter (but the majority of eggs are laid close to the ground between two apposed surfaces) at the end of summer (in Apulia, oviposition was achieved in semi-artificial conditions in October). Hatching occurs in the following spring. The larvae, not very mobile, feed on the sap present in the xylem by sticking their stylets into the plant. Larval development has five instars. Adults appear in April and live until autumn (but they may survive until the successive spring in case of mild winters: Saponari et al. 2014). They are not very active and exhibit a jumping behaviour when they are disturbed.
Although the nymphs live inside the spittle, they can actively crawl over short distances, thus moving from one herbaceous plant to another (Bodino et al., 2017). Adults are much more mobile, both actively and passively. Passive dispersal over great distances is mediated by wind and human activities (transportation by cars can also occur).
Detection and inspection methods
Sampling
Samples for the laboratory should be collected during summertime and be composed of branches or cuttings with (mature) leaves attached. Sampling of young growing shoots briefly after emergence should be avoided because the bacteria may be difficult to detect in the new season’s flush. For small-sized plants, the entire plant can be sent to the laboratory. In the case of detection surveys, it is usually recommended to sample multiple plants and test a pooled sample from the survey site. For example, to test for the presence of X. fastidiosa in olive trees, a laboratory sample may consist of up to 20 g of leaf petioles, corresponding to about 800-900 leaf petioles, while 4 leaf petioles are taken per plant. This would result that up to 200-225 plants are then tested in a single pooled sample.
Vector sampling is considered to be an efficient practice to detect the bacterium in a given area. Currently, sweep nets are commonly used to collect adult insects of P. spumarius (Cruaud et al., 2018; Cornara et al., 2018). Other trapping methods, such as minicage (biocenometers), pitfall traps, sticky traps, aerial suction traps, beat trays and tanglefoot bands have been proven to be less effective than sweep nets. To maximise the likelihood of detection, insect samples should be collected when the adults are abundant in the field and after they have fed on multiple hosts at the end of the summer or after aestivation.
More details about sampling can be found in the EPPO Standard PM 7/24 (EPPO, 2023) and the EFSA Pest survey card (EFSA, 2019a).
Laboratory testing
Given the difficulty of isolating X. fastidiosa, various serological and molecular diagnostic protocols have been validated to be applied directly on plant tissue or insect vectors extracts. Given their lower sensitivity, the use of serological tests is advised only for screening large numbers of symptomatic plants as well as asymptomatic material from an outbreak area or a buffer zone around an outbreak, as the concentration of the bacterium in the infected tissues is likely to be higher. Molecular methods based on the use of PCR, are generally retained to be more sensitive and specific, although the presence of inhibitors may be a problem with some matrices. Their use is recommended in particular for detecting X. fastidiosa in asymptomatic plants, insect vectors and for subspecies assignment directly or by sanger sequencing the amplicons of at least two and up to seven, housekeeping gene fragments (EPPO, 2023). For areas where the pathogen is known to be present, the result of a single test is considered sufficient (EPPO, 2023) but in areas considered to be pest free, for a positive detection to be considered valid, a minimum of two positive screening tests should be undertaken for plant samples. These tests should either differ in the underlying biological principles or in the genomic sequence they target. Remote sensing techniques and hyperspectral imaging methods are becoming more reliable for the early detection of infected trees, particularly for the detection of the pre-visual symptoms (Kumar et al., 2012; Li et al., 2014). In Zarco-Tejada et al. (2018), airborne imaging spectroscopy and thermography revealed the presence of X. fastidiosa in olive trees prior to symptom expression.
Real-time PCR (Harper et al., 2010, erratum 2013) is recommended for detection of X. fastidiosa in vector samples. In order to reduce the number of samples to be tested in the laboratory, it is possible to pool the samples of the same species. For small vectors (e.g. Philaenus), 1–5 heads can be pooled, while for large vectors (e.g. Cicada orni or Aphrophora spp.) a single insect’s head can be used (EPPO, 2023).
More details about laboratory testing can be found in the EPPO Standard PM 7/24 and ISPM 27/DP 25 (EPPO, 2019a; IPPC, 2018).
PATHWAYS FOR MOVEMENT 2020-05-05
X. fastidiosa is unable to spread in the environment autonomously either by contact or by air diffusion. It also appears that X. fastidiosa is not seed-transmitted. Natural spread of X. fastidiosa is mainly ensured by its insect vectors that generally fly short distances (on average up to 100-150 metres) but can be transported by wind over longer distances. The main pathway for entry and spread of X. fastidiosa is the trade, or movement, of plants for planting. Infectious vectors can also be carried over short and long distances on plants, plant parts, or as hitchhikers (i.e. through indirect means, such as clothing or body parts of people, vehicles, onto which they can be passively transported). Fruit, cut flowers, ornamental foliage and wood (not for plant propagation purposes) are considered as unlikely pathways for X. fastidiosa.
PEST SIGNIFICANCE 2020-06-09
Economic impact
In the USA, within the main areas where X. fastidiosa occurs naturally (coastal plains of the Gulf of Mexico), Vitis vinifera and V. labrusca cannot be cultivated because they are rapidly infected due to high rates of natural spread. As a consequence, only selections of V. rotundifolia (muscadine) and specially bred resistant hybrids can be cultivated. The same situation exists throughout tropical America. Pierce's disease is thus a major constraint on grapevine production in the USA and tropical America. In California alone, the value of grapevine losses to Pierce’s disease, including the costs of roguing and replanting diseased vines, is on average of 56.1 million USD/year (wine, table and raisin grapes). Moreover, since another 48.3 million USD are spent by nurseries, citrus growers, and government agencies to limit the populations of H. vitripennis, the estimated cost of Pierce’s disease is approximately of 104.4 million USD per year (Tumber et al., 2014).
By contrast, in peaches, phony disease does not kill trees or cause dieback, but it does significantly reduce the size and number of fruits. An analysis of biophysical effects on peach trees has been made by Anderson & French (1987). The disease was extremely important in the south-eastern USA in the 1940s, when 5-year-old orchards were often found to be 50% affected and older orchards entirely so. However, the efficient control methods now available (insecticides, destruction of infected trees, elimination of wild host plants around orchards) allow a high degree of control, except in areas where incidence is very high.
In citrus, variegated chlorosis has been described by Roistacher as ‘the world's most destructive disease of sweet orange’. It has spread rapidly through large areas of southern Brazil and appears that it cannot be controlled. In a survey made in São Paulo State in June 1990, it was reported that 13 sites out of 920 had infected trees. In a follow-up survey in August 1991, 72 out of the 920 sites were infected (a 5-fold increase in a period of just 14 months). By 2000, it was estimated that the disease affected 34% of the 200 million sweet orange trees (Bové and Ayres, 2008), and some growers in São Paulo state are now planting mangoes instead of citrus.
In olive, the most serious epidemic has developed in Apulia (Southern Italy). The bacterium was diagnosed for the first time in 2013, in the municipal area of Gallipoli (Province of Lecce), and the ‘focus’ of infection was already about 8 000 hectares. Since then the disease has advanced by an average of 2 km a month, and now it has reached the municipal area of Monopoli (Province of Bari), 140 kilometres from Gallipoli. As of 2019, it has been estimated that in the Provinces of Lecce, Brindisi and Taranto approximately 11 million plants were infected, over an area of about 50 000 ha.
Damage concerned olive trees in production as well as nurseries of olive plants and have been estimated at a total of 1.2 billion EUR. An indirect impact concerned the export from Italy of any species of plants susceptible to X. fastidiosa. Finally, the damage caused by X. fastidiosa to the landscape of the ‘Salento’ Peninsula in Apulia and to the Mediterranean cultural heritage associated to olive trees is unquantifiable.
Control
The control of X. fastidiosa relies on different methods and can have different targets: the vector, the bacterium, both the vector and the bacterium, and host plants that may function as reservoirs of X. fastidiosa. Control strategies against insect vectors (mostly P. spumarius in the case of OQDS) should take into account the interactions of the vectors with the pathogen (transmission characteristics), the host plants (of the pathogen and vector) and the environment, as well as the inoculum sources and types of spread (primary and secondary) (Almeida et al., 2005; Lopes et al., 2016).
The management strategies that are detailed below mainly apply to OQDS and are based on currently available information; however, they are likely to evolve as new knowledge becomes available. The objective is to reduce population density of P. spumarius and to avoid its further spread. Primary (from weeds to olives) and secondary spread (among olive trees within and between orchards) is ensured by P. spumarius, and most likely by adult P. spumarius.
The most effective active substances to control adults of P. spumarius on olive trees are neonicotinoids (i.e. acetamiprid) and pyrethroids (deltamethrin) (Dongiovanni et al., 2018a). Application of insect growth regulators (buprofenzin and spirotetramat) against spittlebug nymphs showed very low efficacy. Preliminary data suggest that citrus oil is more effective than pyrethrins, however both products are not persistent. For the control of the juveniles, the neonicotinoids and pyrethroid products cause significant reduction in nymphs on the sprayed vegetation.
In the EPPO region, no antibacterial compounds are routinely applied to perennial crops, except copper, which is unable to cure plants of X. fastidiosa or even to prevent transmission by insects. However, a compound containing zinc, copper and citric acid has been shown to reduce the multiplication of X. fastidiosa in olive tissues (Scortichini et al., 2018).
Another selective and potential control method is the application of particle film technology (e.g. kaolin) to interfere with the vector-host plant selection. When applied on grapes, kaolin particles change the colour of the tree canopy, thus inhibiting landing by sharpshooter vectors and reducing pathogen transmission (Puterka et al., 2003; Tubajika et al., 2007). However, it seems that this method does not work in the case of OQDS.
With regard to biological control, very little is currently known on the use of natural enemies (parasitoids of eggs, nymphs, adults; or entomopathogenic micro-organisms) against X. fastidiosa vectors.
Elimination of weeds within and around olive groves may help in reducing the vector populations. For example, in Apulia tillage performed in winter and spring significantly reduced (almost to zero) the abundance of both P. spumarius and N. campestris on olive trees and ground vegetation.
Sowing of Lolium spp. and Hordeum vulgare as a cover crop in winter decreased P. spumarius juvenile populations.
The most promising control measures against diseases caused by X. fastidiosa rely on the use of resistant germplasm. Various degrees of resistance, tolerance and susceptibility have been observed in economically relevant crops such as grape, citrus, almond, and more recently, olive. In this last case extended surveys and experimental infectivity studies identified cv. Leccino as being tolerant to X. fastidiosa subsp. pauca ST53 infections based on lower incidence, lower bacterial levels, and symptom severity when compared to cv. Ogliarola salentina (Boscia et al., 2017). It is also hoped that tolerance or resistance traits can also be found in other olive varieties: one of these is the cultivar FS-17 also known as ‘Favolosa’. In California, grape selections carrying a genetic trait that confers resistance/tolerance to Pierce’s disease of Vitis spp. have been released to grapevine nurseries for propagation and resistant varieties should become soon available for commercial use (Walker et al., 2017). Moreover, transgenic grapevine rootstocks, expressing a pear polygalacturonase inhibitory protein (PGIP) or a chimeric antimicrobial protein (CAP), have showed resistance to X. fastidiosa. The search for resistant germplasm also continues in the case of Prunus and Citrus spp.
Finally, control of X. fastidiosa also consists in minimizing other sources of stress to the host plant (e.g. drought, overproduction, other diseases). The effect of some agricultural practices on Xylella infections, such as pruning, irrigation and fertilisation, have also been investigated with encouraging results.
Phytosanitary risk
From 1981 to 2017, X. fastidiosa was included in the EPPO A1 List of pests recommended for regulation as quarantine pests, but following its introduction in the EPPO region, it was transferred to the EPPO A2 List in 2017. In 2019, X. fastidiosa was included in the list of priority pests of the European Union, in the framework of its new plant health legislation (EU, 2019). The introduction of X. fastidiosa subsp. pauca strain ST53 and different ST of X. fastidiosa subsp. multiplex in the EPPO region represents a high risk to economically important crops and to the environment. In particular, the grapevine strain of X. fastidiosa had and still has the potential to kill large numbers of grapevines and to make areas unfit for growing V. vinifera. Its North American vectors do not occur in the EPPO region, but vector capacity is so non-specific that one could certainly expect European Cicadellinae (e.g. Cicadella viridis) or Cercopidae to transmit the bacterium if introduced. The main threat in the long term is that X. fastidiosa could become established in natural vegetation which would then act as a reservoir for infection of vineyards.
Models have been used to estimate the potential distribution of X. fastidiosa, and have revealed that the following areas in Southern Europe and the Mediterranean area were climatically suitable for the establishment of X. fastidiosa and were more particularly at risk: Atlantic regions of Southern Portugal and Southwestern Spain, coastal areas in Southern France, Italy and Croatia as well as all their islands and archipelagos. Moreover subsp. multiplex could possibly establish further north in the EU compared to other subspecies (EFSA, 2019b). However, as the potential disease distribution may depend on the biology of its potential vectors, it is accordingly rather difficult to assess.
The presence in the EPPO region of different populations and subspecies of the bacterium may allow the appearance of recombining strains, resulting from crosses between the different subspecies. These events may increase the risk of appearance of new pathogenic strains that are more virulent or pathogenic to new hosts (Vanhove et al., 2019). The likelihood of such crosses between different strains could be favoured by the existence of an overlapping host range. For example, it has been determined that in the European Union territory, out of the 43 plant species reported as host of subspecies pauca, 23% were also shown to be host of another subspecies, most frequently subsp. multiplex, and that 35% may be host for all the three subspecies (European Commission, 2020).
PHYTOSANITARY MEASURES 2020-05-05
The most effective method for preventing the introduction and spread of X. fastidiosa into uninfected areas is to impose strict phytosanitary measures and perform inspections (EPPO, 2016b) on imported host plant material, as well as to survey the health status of potential host plants and vectors, in particular in orchards and nurseries (EPPO, 2016c). In this respect, correct and timely disease identification is crucial for the appropriate application of any phytosanitary measure. Rapid detection (and destruction) of new foci of infection are also of the greatest importance.
Within the EPPO region, phytosanitary regulations are in place to prevent the entry and spread of X. fastidiosa. For the EU Member States, phytosanitary measures are detailed in the Decision (EU) 2015/789 (EU, 2015).
Considering the serious threat that X. fastidiosa represents for grapevine and citrus cultivation, prohibitions to import plants for planting of citrus, grapevine and coffee plants from third countries where X. fastidiosa occurs have been imposed by many EPPO member countries. In addition, several countries require that host plants for planting from infected third countries are either grown in a pest free area, or under protected conditions with inspection, sampling and testing for the absence of the bacterium prior to their export. For certain host plants (e.g. Vitis plants for planting), hot water treatment (50°C for 45 min) can be considered effective to sanitize plants for planting coming from an infected area (EFSA, 2015b). Plants for planting can also be grown under complete physical isolation (EPPO, 2016d) to exclude insect vectors.
Countries are also recommended to carry out regular surveys to verify the absence or presence of X. fastidiosa on their territory. Within the European Union territory, specific annual surveys should be carried out by all Member States and lists of specified host plants have been defined to that effect (European Commission, 2015, 2020; EFSA, 2019a). Detailed information regarding the disease spread capacity is of course fundamental when determining the extent of the area to survey. In this respect, the use of spread models could facilitate the decision-making process. For example, a model has been used to determine the disease spread within an olive orchard. It was estimated that the median short-distance spread was of approximately 150 m per year and that the median long-distance spread was of approximately 10 km per year (EFSA, 2019b). However, the above values may change according to several parameters, such as the density, spatial distribution and number of different of host plants species, abundance, mobility and biological characteristics of insect vectors.
In the event of a new outbreak of X. fastidiosa, eradication or containment measures should be applied. These include the delimitation of infected and buffer zones where appropriate official measures are applied with varying intensity according to the zone concerned. These measures include the destruction of infected plants and potential host plants located in their vicinity, specific surveys (including intensive sampling and testing), restrictions on the movement of host plants, as well as appropriate control measures against the vectors and plants that may host those vectors. So far, it has to be recognized that no eradication attempts have been successful in outdoor conditions, and in areas where the disease has established.
REFERENCES 2023-09-07
Almeida RPP, Blua MJ, Lopes JR, Purcell AH (2005) Vector transmission of Xylella fastidiosa: applying fundamental knowledge to generate disease management strategies. Annals of the Entomological Society of America 98, 775-786.
Anderson PC, French WJ (1987) Biophysical characteristics of peach trees infected with phony peach disease. Physiological and Molecular Plant Pathology 31, 25-40.
Berisha B, Chen YD, Zhang GY, Xu BY, Chen TA (1998) Isolation of Pierce's disease bacteria from grapevines in Europe. European Journal of Plant Pathology 104, 427-433.
Bodino N, Plazio E, Cavalieri V, Dongiovanni E, Ripamonti M, Volani S, Gilioli G, Fumarola G, Di Carolo M, Porcelli F, Bosco D (2017) Host-plant association and host-shifting of nymphs and adults of Philaenus spumarius L. in Italian olive orchards. In: Proceedings of the 3rd Hemipteran-plant interactions symposium (HPIS), Madrid, Spain, 4-8 June 2017, 36 pp.
Boscia D, Altamura G, Ciniero A, Di Carolo M, Dongiovanni C, Fumarola G, Giampetruzzi A, Greco P, La Notte P, Loconsole G, Manni F, Melcarne G, Montilon V, Morelli M, Murrone N, Palmisano F, Pollastro P, Potere O, Roseti V, Saldarelli P, Saponari A, Saponari M, Savino V, Silletti MR, Specchia F, Susca L (2017) Resistance to Xylella fastidiosa in different olive cultivars. Informatore Agrario 73, 59-63.
Bové JM, Ayres AJ (2008) Etiology of three recent diseases of citrus in São Paulo State: Sudden death, variegated chlorosis and huanglongbing. IUBMB Life 59(4-5), 346-354.
Bull CT, De Boer SH, Denny TP, Firrao G, Fischer-Le Saux M, Saddler GS, Scortichini M, Stead DE, Takikawa Y (2012) List of new names of plant pathogenic bacteria (2008-2010). Journal of Plant Pathology 94, 21-27.
Cavalieri V, Dongiovanni C, Tauro D, Altamura G, Di Carolo M, Fumarola G, Saponari M, Bosco D (2018) Transmission of the CODIRO strain of Xylella fastidiosa by different insect species. In: Proceedings of the XI European Congress of Entomology, Naples, Italy, 2-6.
Chu F‐LE, Volety AK (1997) Disease processes of the parasite Perkinsus marinus in eastern oyster Crassostrea virginica: minimum doses for infection initiation, and interaction of temperature, salinity and infective cell dose. Diseases of Aquatic Organisms 28, 61-68.
Coletta-Filho H, Francisco CS, Lopes JRS, de Oliveira AF, da Silva LF (2016) First report of olive leaf scorch in Brazil, associated with Xylella fastidiosa subsp. pauca. Phytopathologia Mediterranea 55, 130-135.
Cornara D, Saponari M, Zeilinger AR, de Stradis A, Boscia D, Loconsole G, Bosco D, Martelli GP, Almeida RP, Porcelli F (2017a) Spittlebugs as vectors of Xylella fastidiosa in olive orchards in Italy. Journal of pest science 90(2), 521-530.
Cornara D, Cavalieri V, Dongiovanni C, Altamura G, Palmisano F, Bosco D, Porcelli F, Almeida RP, Saponari M (2017b) Transmission of Xylella fastidiosa by naturally infected Philaenus spumarius (Hemiptera, Aphrophoridae) to different host plants. Journal of Applied Entomology 141, 80-87.
Cornara D, Bosco D, Fereres A (2018) Philaenus spumarius: when an old acquaintance becomes a new threat to European agriculture. Journal of Pest Science 91, 957-972.
Cruaud A, Gonzalez A, Godefroid M, Nidelet S, Streito J, Thuillier J, Rossi JP, Santoni S, Rasplus J (2018) Using insects to detect, monitor and predict the distribution of Xylella fastidiosa: a case study in Corsica. Scientific Reports 8(1). https://doi.org/10.1038/s41598-018-33957-z
Denancé N, Legendre B, Briand M, Olivier V, de Boisseson C, Poliakoff F, Jacques MA (2017) Several subspecies and sequence types are associated with the emergence of Xylella fastidiosa in natural settings in France. Plant Pathology 66, 1054-1064.
Denancé N, Briand M, Gaborieau R, Gaillard S, Jacques MA (2019) Identification of genetic relationships and subspecies signatures in Xylella fastidiosa. BMC genomics 20, 239. https://doi.org/10.1186/s12864-019-5565-9
Di Serio F, Bodino N, Cavalieri V, Demichelis S, Di Carolo M, Dongiovanni C, Fumarola G, Gilioli G, Guerrieri E, Picciotti U, Plazio E, Porcelli F, Saladini M, Salerno M, Simonetto A, Tauro D, Volani S, Zicca S, Bosco D (2019) Collection of data and information on biology and control of vectors of Xylella fastidiosa. EFSA supporting publication 1628E, 102 pp. https://doi.org/10.2903/sp.efsa.2019.EN-1628
Dohm DJ, O’Guinn ML, Turrell MJ (2002) Effect of environmental temperature on the ability of Culex pipiens (Diptera: Culicidae) to transmit West Nile virus. Journal of Medical Entomology 39, 221-225.
Dongiovanni C, Altamura G, Di Carolo M, Fumarola G, Saponari M, Cavalieri V (2018a) Evaluation of efficacy of different insecticides against Philaenus spumarius L., vector of Xylella fastidiosa in olive orchards in Southern Italy, 2015-17. Arthropod Management Tests 43(1), 1-4. https://doi.org/10.1093/amt/tsy034
Dongiovanni C, Cavalieri V, Bodino N, Tauro D, Di Carolo M, Fumarola G, Altamura G, Lasorella C, Bosco D (2018b) Plant selection and population trend of spittlebug immatures (Hemiptera: Aphrophoridae) in olive groves of the Apulia Region of Italy. Journal of Economic Entomology 112(1), 67-74.
EFSA (2015a) Scientific Opinion on the risks to plant health posed by Xylella fastidiosa in the EU territory, with the identification and evaluation of risk reduction options. EFSA Journal 13(1), 3989, 262 pp. https://doi.org/10.2903/j.efsa.2015.3989
EFSA (2015b) Scientific Opinion on hot water treatment of Vitis sp. for Xylella fastidiosa. EFSA Journal 13(9), 4225, 10 pp. https://doi.org/10.2903/j.efsa.2015.4225
EFSA (2018) Scientific Opinion on the updated pest categorisation of Xylella fastidiosa. EFSA Journal 16(7), 5357, 61 pp. https://doi.org/10.2903/j.efsa.2018.5357
EFSA (2019a) Pest survey card on Xylella fastidiosa. EFSA Supporting publication EN-1667. https://doi.org/10.2903/sp.efsa.2019.EN-1667
EFSA (2019b) Update of the Scientific Opinion on the risks to plant health posed by Xylella fastidiosa in the EU territory. EFSA Journal 17(5), 5665, 200 pp. https://doi.org/10.2903/j.efsa.2019.5665
EFSA (2019c) Scientific Opinion on the pest categorisation of non-EU Cicadomorpha vectors of Xylella spp. EFSA Journal 17(6), 5736. https://doi.org/10.2903/j.efsa.2019.5736
EFSA (2020) Update of the Xylella spp. host plant database – systematic literature search up to 30 June 2019. EFSA Journal 18(4), 6114, 61 pp. https://doi.org/10.2903/j.efsa.2020.6114
EPPO (1998) EPPO Reporting Service 1998/157. https://gd.eppo.int/reporting/article-3666
EPPO (2016b) PM 3/81 (1) Inspection of consignments for Xylella fastidiosa. EPPO Bulletin 46, 395-406. https://doi.org/10.1111/epp.12325
EPPO (2016c) PM 3/82 (1) Inspection of places of production for Xylella fastidiosa. EPPO Bulletin 46, 407-418. https://doi.org/10.1111/epp.12328
EPPO (2016d) PM 5/8 (1) Guidelines on the phytosanitary measure ‘Plants grown under complete physical isolation’. EPPO Bulletin 46, 421-423. https://doi.org/10.1111/epp.12340
EPPO (2023) PM 7/24 (5) Diagnostic standard for Xylella fastidiosa. EPPO Bulletin 53(2), 205-276.
European Commission (2015) Guidelines for the survey of Xylella fastidiosa (Wells et al.) in the Union territory. Directorate-General for Health and Food Safety, Brussels. https://ec.europa.eu/food/sites/food/files/plant/docs/ph_biosec_legis_guidelines_xylella-survey.pdf
European Commission (2020) Commission database of host plants found to be susceptible to Xylella fastidiosa in the Union territory. https://ec.europa.eu/food/plant/plant_health_biosecurity/legislation/emergency_measures/xylella-fastidiosa/susceptible_en (last accessed 2020-03)
EU (2015) Commission implementing decision (EU) 2015/789 of 18 May 2015 as regards measures to prevent the introduction into and the spread within the Union of Xylella fastidiosa (Wells et al.). Official Journal of the European Union 125, 36-53.
EU (2019) Commission delegated regulation (EU) 2019/1702 pf 1 August 2019 supplementing Regulation (EU) 2016/2031 of the European Parliament and of the Council by establishing the list of priority pests. Official Journal of the European Union 206, 8-10.
Feil H, Purcell AH (2001) Temperature-dependent growth and survival of Xylella fastidiosa in vitro and in potted grapevines. Plant Disease 85, 1230-1234.
Frazier NW (1944) Phylogenetic relationship of the nine known leaf-hopper vectors of Pierce’s disease of grape. Phytopathology 34, 1000-1001.
Freitag JH (1951) Host range of the Pierce's disease virus of grapes as determined by insect transmission. Phytopathology 41, 10.
Güldür ME, Çağlar BK, Castellano MA, Ünlü L, Güran S, Yılmaz MA, Martelli GP (2005) First report of almond leaf scorch in Turkey. Journal of Plant Pathology 87, p 246.
Habib W, Nigro F, Gerges E, Jreijiri F, Al Masri Y, El Riachy M, Choueiri E (2016) Xylella fastidiosa does not occur in Lebanon. Journal of Phytopathology 164, 395-408.
Haelterman RM, Tolocka PA, Roca ME, Guzman FA, Fernandez FD, Otero ML (2015) First presumptive diagnosis of Xylella fastidiosa causing olive scorch in Argentina. Journal of Plant Pathology 97, p 393.
Harper SJ, Ward LI, Clover GRG (2010) Development of LAMP and real-time PCR methods for the rapid detection of Xylella fastidiosa for quarantine and field applications. Phytopathology 100, 1282-1288.
Hendson M, Purcell AH, Chen D, Smart C, Guilhabert M, Kirkpatrick B (2001) Genetic diversity of Pierce's disease strains and other pathotypes of Xylella fastidiosa. Applied and Environmental Microbiology 67(2), 895-903.
Hernandez-Martinez R, de la Cerda KA, Costa HS, Cooksey DA, Wong FP (2007) Phylogenetic relationships of Xylella fastidiosa strains isolated from ornamentals in southern California. Phytopathology 97, 857-864.
Hill BL, Purcell AH (1995) Acquisition and retention of Xylella fastidiosa by an efficient vector, Graphocephala atropunctata. Phytopathology 85, 209-212.
Hill BL, Purcell AH (1997) Populations of Xylella fastidiosa in plants required for transmission by an efficient vector. Phytopathology 87, 1197-1201.
Hopkins D, Purcell A (2002) Xylella fastidiosa: cause of Pierce’s disease of grapevine and other emergent diseases. Plant Disease 86, 1056-1066.
IPPC (2018) ISPM 27 Diagnostic protocols for regulated pests. DP 25: Xylella fastidiosa. FAO, Rome, 32 pp. https://www.ippc.int/static/media/files/publication/en/2018/09/DP_25_2018_Xylellafastidiosa_2018-09-21.pdf
Krugner R (2011) Evaluation of pathogenicity and insect transmission of Xylella fastidiosa strains to olive Plants. In: California Olive Committee, Final & Interim Research Reports 2010, Compiled by MW Johnson, 3-11.
Kyrkou I, Pusa T, Ellegaard-Jensen L, Sagot M-F, Hansen LH (2018) Pierce’s disease of grapevines: A review of control strategies and an outline of an epidemiological model. Frontiers in Microbiology 9, 2141. https://doi.org/10.3389/fmicb.2018.02141
Kumar A, Lee WS, Ehsani RJ, Albrigo LG, Yang C, Mangan RL (2012) Citrus greening disease detection using aerial hyperspectral and multispectral imaging techniques. Journal of Applied Remote Sensing 6(1), 063542. https://doi.org/10.1117/1.JRS.6.063542
Li H, Lee WS, Wang K, Ehsani R, Yang C (2014) ‘Extended spectral angle mapping (ESAM)’ for citrus greening disease detection using airborne hyperspectral imaging. Precision Agriculture 15, 162-183.
Lopes JRS, Daugherty MP, Almeida RPP (2010) Strain origin drives virulence and persistence of Xylella fastidiosa in alfalfa. Plant Pathology 59, 963-971.
Lopes JRS, Krugner R and Brown J (2016) Transmission ecology and epidemiology of the citrus variegated chlorosis strain of Xylella fastidiosa. In: Brown JK (ed.). Vector-mediated Transmission of Plant Pathogens. APS Press, pp. 195-208.
Maiden MC, Bygraves JA, Feil E, Morelli G, Russell JE, Urwin R, Zhang Q, Zhou J, Zurth K, Caugant DA, Feavers IM, Achtman M, Spratt BG (1998) Multilocus sequence typing: a portable approach to the identification of clones within populations of pathogenic microorganisms. Proceedings of the National Academy of Sciences of the United States of America 95, 3140-3145.
Martelli GP (2016) Il punto su Xylella fastidiosa e disseccamento rapido dell’olivo. L’informatore Agrario 24, 40-48.
Noordijk J, den Bieman CFM, de Haas MS and Colijn Ed O (2019) Xyleemzuigende cicaden, potentiële vectoren van Xylella fastidiosa, rondom boomkwekerijen en glastuinbouw. EIS Kenniscentrum Insecten, Leiden. NVWA, afdeling NRC, EIS2019-06. Available online at: https://www.eisnederland.nl/DesktopModules/Bring2mind/DMX/Download.aspx?command=core_download&entryid=801&language=nl-NL&PortalId=4&TabId=563
Nunney L, Ortiz B, Russell SA, Ruiz Sanchez R, Stouthamer R (2014) The complex biogeography of the plant pathogen Xylella fastidiosa: genetic evidence of introductions and subspecific introgression in Central America. PLoS ONE 9, e112463.
Nunney L, Azad H, Stouthamer R (2019) An experimental test of the host-plant range of nonrecombinant strains of North American Xylella fastidiosa subsp. multiplex. Phytopathology 109(2), 294-300.
Purcell AH (1980) Almond leaf scorch: leafhopper and spittlebug vectors. Journal of Economic Entomology 73, 834-838.
Purcell AH (1989) Homopteran transmission of xylem-inhabiting bacteria. In: Harris KF (ed). Advances in disease vector research. Vol 6. Springer, New York, USA, 243-266.
Purcell AH, Finlay AH (1979) Evidence for noncirculative transmission of Pierce’s disease bacterium by sharpshooter leafhoppers. Phytopathology 69, 393-395.
Puterka GJ, Reinke M, Luvisi D, Ciomperik MA, Bartels D, Wendel L, Glenn DM (2003) Particle film, surround WP, effects on glassy-winged sharpshooter behavior and its utility as a barrier to sharpshooter infestations in grape. Online. Plant Health Progress https://doi.org/10.1094/PHP-2003-0321-01-RS
Redak RA, Purcell AH, Lopes JR, Blua MJ, Mizell Iii, RF and Andersen PC (2004) The biology of xylem fluid-feeding insect vectors of Xylella fastidiosa and their relation to disease epidemiology. Annual Reviews in Entomology 49(1), 243-270.
Saponari M, Boscia D, Nigro F, Martelli GP (2013) Identification of DNA sequences related to Xylella fastidiosa in oleander, almond and olive trees exhibiting leaf scorch symptoms in Apulia (Southern Italy). Journal of Plant Pathology 95, 659-668.
Saponari M, Loconsole G, Cornara D, Yokomi RK, De Stradis A, Boscia D, Bosco D, Martelli GP, Krugner R, Porcelli F (2014) Infectivity and transmission of Xylella fastidiosa by Philaenus spumarius (Hemiptera: Aphrophoridae) in Apulia, Italy. Journal of Economic Entomology 107(4), 1316-1319.
Scally M, Schuenzel EL, Stouthamer R, Nunney L (2005) A multilocus sequence type system for the plant pathogen Xylella fastidiosa, and the relative contribution of recombination versus point mutation to clonal diversity. Applied and Environmental Microbiology 71, 8491-8499.
Scortichini M, Chen J, De Caroli M, Dalessandro G, Pucci N, Modesti V, L’Aurora A, Petriccione M, Zampella L, Mastrobuoni F, Migoni D, Del Coco L, Girelli CR, Piacente F, Cristella N, Marangi P, Laddomada F, Di Cesare M, Cesari G, Fanizzi FP, Loreti S (2018) A zinc, copper and citric acid biocomplex shows promise for control of Xylella fastidiosa subsp. pauca in olive trees in Apulia region (southern Italy). Phytopathologia Mediterranea 57, 48-72.
Severin HHP (1950) Spittle-insect vectors of Pierce’s disease virus: II. Life history and virus transmission. Hilgardia 19, 357-382.
Su T, Mulla MS (2001) Effects of temperature on development, mortality, mating and blood feeding behavior of Culiseta incidens (Diptera: Culicidae). Journal of Vector Ecology 26, 83-92.
Su CC, Chang CJ, Chang CM, Shih HT, Tzeng KC, Jan FJ, Kao CW, Deng, WL (2013) Pierce's disease of grapevines in Taiwan: isolation, cultivation and pathogenicity of Xylella fastidiosa. Journal of Phytopathology 161(6), 389-396.
Su CC, Deng WL, Jan FJ, Chang CJ, Huang H, Shih HT, Chen J (2016) Xylella taiwanensis sp. nov., causing pear leaf scorch disease. International journal of systematic and evolutionary microbiology 66(11), 4766-4771.
Temsah M, Hanna I, Saad A (2015) First report of Xylella fastidiosa associated with oleander leaf scorch in Lebanon. Journal of Crop Protection 4, 131-137.
Tumber KP, Alston JM, Fuller KB (2014) Pierce’s disease costs California $104 million per year. California Agriculture 68(1-2), 20-29.
Tubajika KM, Civerolo EL, Puterka GJ, Hashimd JM, Luvisid DA (2007) The effects of kaolin, harpin, and imidacloprid on development of Pierce’s disease in grape. Crop Protection 26, 92-99.
Walker A, Cantu D, Riaz S, Agüero C (2017) Molecular breeding support for the development of Pierce’s disease resistant winegrapes. In: Proceedings of Pierce’s Disease Research Symposium, 137-147.
Vanhove M, Adam C., Retchless AC, Sicard A, Rieux A, Coletta-Filho HD, De La Fuente L, Stenger DC, Almeida RPP (2019) Genomic diversity and recombination among Xylella fastidiosa subspecies. Applied and Environmental Microbiology 85, e02972-18. https://doi.org/10.1128/AEM.02972-18
Weaver CR, King DR (1954) Meadow spittlebug. Research Bulletin no. 741. Ohio Agricultural Experiment Station, Wooster, OH.
Young DA (1968) Taxonomic study of the Cicadellinae (Homoptera: Cicadellidae). Part 1. Proconinii. United States National Museum Bulletin 261. Washington, DC, USA.
Young DA (1977) Taxonomic study of the Cicadellinae (Homoptera: Cicadellidae). Part 2. New World Cicadellini and the genus Cicadella. North Carolina Agricultural Experiment Station Bulletin 239. Raleigh, North Carolina, USA.
Yuan X, Morano L, Bromley R, Spring-Pearson S, Stouthamer R, Nunney L (2010) Multilocus sequence typing of Xylella fastidiosa causing Pierce’s disease and oleander leaf scorch in the United States. Phytopathology 100, 601-611.
Yurtsever S (2000) On the polymorphic meadow spittlebug, Philaenus spumarius (L.) (Homoptera: Cercopidae). Turkish Journal of Zoology 24, 447-459.
Zarco-Tejada PJ, Camino C, Beck PSA, Calderon R, Hornero A, Hernández-Clemente R Kattenborn T, Montes-Borrego M, Susca L, Morelli M, Gonzalez-Dugo V, North PRJ, Landa BB, Boscia D, Saponari M, Navas-Cortes JA (2018) Previsual symptoms of Xylella fastidiosa infection revealed in spectral plant-trait alterations. Nature Plants 4(7), 432.
CABI and EFSA resources used when preparing this datasheet
CABI (2020) Invasive Species Compendium. Datasheet on Xylella fastidiosa (Pierce's disease of grapevines). https://www.cabi.org/isc/datasheet/57195
EFSA (2019) Pest survey card on Xylella fastidiosa. https://www.efsa.europa.eu/en/supporting/pub/en-1667
ACKNOWLEDGEMENTS 2020-05-05
This datasheet was extensively revised in 2020 by Giuseppe Surico and Guido Marchi from the ‘Dipartimento di Scienze e Tecnologie Agrarie, Alimentari, Ambientali e Forestali (DAGRI), Sez. Patologia ed entomologia, Università di Firenze, Firenze, Italy’. Their valuable contribution is gratefully acknowledged.
How to cite this datasheet?
Datasheet history 2020-05-05
This datasheet was first published in the EPPO Bulletin in 1989 and revised in the two editions of 'Quarantine Pests for Europe' in 1992 and 1997. It is now maintained in an electronic format in the EPPO Global Database. The sections on 'Identity', ‘Hosts’, and 'Geographical distribution' are automatically updated from the database. For other sections, the date of last revision is indicated on the right.
CABI/EPPO (1992/1997) Quarantine Pests for Europe (1st and 2nd edition). CABI, Wallingford (GB).
EPPO (1989) Data sheets on quarantine organisms No. 166, Xylella fastidiosa. Bulletin OEPP/EPPO Bulletin 13(1), 677-682.
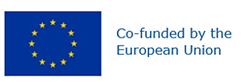