Stenocarpella maydis(DIPDMA)
EPPO Datasheet: Stenocarpella maydis
IDENTITY
Authority: (Berkeley) Sutton
Taxonomic position: Fungi: Ascomycota: Pezizomycotina: Sordariomycetes: Diaporthomycetidae: Diaporthales: Diaporthaceae
Other scientific names: Diplodia maydis (Berkeley) Saccardo, Diplodia zeae-maydis Mekhtieva, Diplodia zeae (Schweinitz) Léveillé, Dothiora zeae (Schweinitz) Bennett, Hendersonia zeae (Léveillé) Hazslin, Macrodiplodia zeae (Schweinitz) Petrák & Sydow, Sphaeria maydis Berkeley
Common names in English: leaf spot of maize, seedling blight of maize, stalk rot of maize, white ear rot of maize
view more common names online...
EPPO Categorization: A2 list
view more categorizations online...
EPPO Code: DIPDMA
HOSTS 2023-11-30
Stenocarpella maydis has a narrow host range with Zea mays as the main host. Bamboos (Arundinaria species (Sutton & Waterston, 1996) and Bambusa species (Farr & Rossman, 2022)) have also been reported as hosts.
Host list: Arundinaria sp., Bambusa sp., Zea maysGEOGRAPHICAL DISTRIBUTION 2023-11-30
Stenocarpella maydis is distributed in almost all regions of the world. It can be found in Africa, Asia, America and Oceania. In Europe, it is reported in the Czech Republic (EPPO, 2023), Serbia (EPPO, 2023) and Spain (de la Riva et al., 1994). In some EPPO countries it has only been intercepted (e.g. Bulgaria), and in others it has been detected in the past but is no longer present (e.g. Austria) (EPPO, 2023).
Africa: Congo, Congo, Democratic republic of the, Eswatini, Ethiopia, Ghana, Kenya, Malawi, Nigeria, South Africa, Tanzania, Uganda, Zambia, Zimbabwe
Asia: China (Gansu, Guangdong, Guangxi, Guizhou, Henan, Hubei, Jiangsu, Jilin, Liaoning, Shanxi, Sichuan, Yunnan), India (Sikkim), Iran, Korea, Republic, Nepal, Pakistan, Philippines, Taiwan, Thailand
North America: Canada (Ontario), Mexico, United States of America (Alabama, Arkansas, California, Connecticut, Delaware, Florida, Georgia, Illinois, Indiana, Iowa, Kansas, Kentucky, Louisiana, Maine, Maryland, Massachusetts, Michigan, Minnesota, Mississippi, Missouri, Nebraska, New Jersey, New York, North Carolina, North Dakota, Ohio, Oklahoma, Pennsylvania, Rhode Island, South Carolina, South Dakota, Tennessee, Texas, Virginia, Washington, West Virginia, Wisconsin)
Central America and Caribbean: Belize, El Salvador, Guatemala, Honduras
South America: Argentina, Bolivia, Brazil (Minas Gerais, Parana, Rio Grande do Sul, Santa Catarina), Colombia, Ecuador
Oceania: Australia (New South Wales), New Zealand
BIOLOGY 2023-11-30
Stenocarpella maydis is necrotrophic, exhibiting a parasitic phase in the developing plant and saprophytic phase in the crop residue. S. maydis overwinters as viable pycnidia and mycelium on maize debris in the soil (Flett et al., 1992) or on seed. Under warm, moist conditions spores are extruded from pycnidia in long cirrhi and disseminated by wind and rain and, probably by insects.
Maize plants are infected primarily through the crown, mesocotyl and roots and, occasionally, at the nodes between crown and ear. Following this, stalks are invaded. The development of the stalk rot phase is favoured by dry weather early in the growing season, followed by extended periods of rainfall shortly after silking. In stalk infections, injury to the vascular system disrupts translocation and, consequently, reduces grain size.
McNew (1937) recovered S. maydis from field soil planted with maize which suggests it can survive in the soil. Luna et al. (2017) suggest survival in the soil can be for up to 17 months.
Unbalanced fertility, low potassium, poor drainage, mechanical and insect damage, cultivar, and planting density all influence disease severity. The ear and grain rotting phase is similarly favoured by above-normal rainfall from silking to harvest, ears being most susceptible during the weeks after silking. Invasion of the ear is usually by way of the shank. Hybrids with poor husk coverage or thin pericarps are often highly susceptible.
For more information, see Koehler (1960), Dhanraj (1966), Sutton & Waterston (1966), Christensen & Wilcoxson (1967), Walker (1969), Shurtleff (1980).
DETECTION AND IDENTIFICATION 2023-11-30
Symptoms
Seedlings
Infected seed gives rise to pre-emergence death in cold soils or blighted seedlings in warmer soils. Seedlings develop brown, cortical lesions on the internode between the scutellum and coleoptile, and the seminal roots are frequently destroyed.
Stalk rot
Symptoms do not usually appear until several weeks after silking, and generally arise following root infection. Oval, irregular or elongate, single or confluent lesions, 1-10 cm long, with pale cream-brown centres and indeterminate darker borders are frequently associated with stalk rot infection. Leaves wilt, become dry and appear greyish-green, the symptoms resembling frost damage. Affected plants may die suddenly. The green colour of the internodes fades and they become brown to straw-coloured, spongy and easily crushed. The pith disintegrates and becomes discoloured, with only the vascular bundles remaining intact. Dark, sub-epidermal pycnidia may be seen clustered near the nodes, and white fungal growth may also be present on the surface (Sutton & Waterston, 1966; Christensen & Wilcoxson, 1967; Walker, 1969; Shurtleff, 1980).
Ear rot
Infection usually begins at the ear base, moving up from the shank. If infection occurs within 2 weeks after silking, the entire ear turns greyish-brown, shrunken and completely rotten and light. Alternatively, early infections result in bleached or straw-coloured husks. Lightweight ears usually stand upright with inner husks adhering tightly to one another or to the ear because of mycelial growth between them. Black pycnidia may be scattered on husks, floral bracts and the sides of kernels. Late-infected ears show no external symptoms, but when ears are broken and grains removed, a white mould is commonly found growing between the grains whose tips are discoloured. For more information, see Sutton & Waterston (1966), Christensen & Wilcoxson (1967), Walker (1969), Shurtleff (1980).
Morphology
Since a number of primary and secondary fungi may be present, microscopic observation of fruiting bodies is recommended.
Pycnidia are immersed, spherical to subglobose, 150-300 µm in diameter, with multicellular walls and a circular protruding papillate ostiole, 30-40 µm in diameter. Conidia in S. maydis are straight, curved or irregular, 1 (0-2) septate, smooth-walled and pale-brown with rounded or truncated ends, 5-8 x 15-34 µm Sutton & Waterston (1966).
Detection and inspection methods
Visual examination in a site of production is not an appropriate method for the detection of S. maydis, and samples should be sent directly for laboratory testing (EPPO, 2021).
Seed infested with S. macrospora show discoloration and are shriveled, moldy and may be rotten when heavily infected. These symptoms are not specific to this pest as may fungal infestations show similar symptoms. Seeds may be infested asymptomatically.
Isolation of S. maydis is possible from the infected kernels, seed, cob and stalk rind or pith and root tissues (Flett & Wehner, 1991; Flett et al., 1992).
Seeds of maize should be placed on 1% malt agar and incubated at 20°C for 7 days. Subsequent microscopical observation should then reveal the presence of the fungi. The Japanese Plant Protection Service proposed a procedure which required less time by removing the outer layers of the seeds halfway through the incubation period, with subsequent microscopic examination (Dai et al., 1987).
Stenocarpella maydis and S. macrospora can be collectively detected using a genus-specific primer (Xia & Achar, 2001). A molecular biology approach based on real-time PCR is an easy, fast, and sensitive test for specific targets compared to conventional methods of diagnosis. Barros et al. (2014) and Pinto et al. (2022) have developed and validated real-time PCR tests for S. maydis.
PATHWAYS FOR MOVEMENT 2023-11-30
International spread by S. maydis will most probably take place through infected maize seed for planting and intended for animal feed. Studies showed that the mycelium of S. maydis is present in the endosperm and embryo of maize seeds (Zad & Ale Agha, 1985). EFSA (2022) note that as S. maydis can survive in host plant debris in soil, therefore soil and other growing media may be a pathway for movement. Non-host plants with infected debris in the growing media may be a pathway for entry. EFSA (2022) also note that mycelium, pycnidia and conidia may also be a contaminant other substrates (e.g. straw, used machinery and equipment). Natural spread of the fungi can be regarded as rather limited.
PEST SIGNIFICANCE 2023-11-30
Economic impact
Stenocarpella maydis has been shown to cause between 5 and 37% reduction in germination (Nwigwe, 1974), as well as being a serious pathogen of maturing plants. Stalk and grain rots are universally important and among the most destructive diseases of maize throughout the world. In most cases, rots are caused by a complex of several species of fungi and bacteria, rather than by a single species, so it is difficult to assess the loss due to S. maydis alone.
Losses due to stalk and grain rots vary from season to season and between regions. Baer et al. (2021) detail that S. maydis can affect yield with losses varying from 1-2 % to as high as 80 %. In the USA, 5-20 % annual yield losses were estimated due to stalk rot and lodging (Christenson & Wilcoxsen, 1966). Differences in grain weight between stalk-rotted and healthy plants in a naturally infected field ranged from 0 to 26.2%. Relating these data to disease incidence in Illinois, state-wide losses were estimated to be 8.6% (Hooker and Britton, 1962). In artificial conditions, Chambers (1988) found yield losses (grain weight per plant) as high as 97% from Diplodia ear rot inoculations made 10 days after silking.
Grain infected by S. maydis has been reported to cause Diplodiosis when fed to cattle and sheep. Different toxic metabolites have been isolated from S. maydis including diplodiatoxin , dipmatol, diplonine and chaetoglobosins K and L (Steyn et al., 1972; Wicklow et al., 2011; Snyman et al., 2011; Masango et al., 2015).
Control
A balanced nutritional status of the soil, reducing stress, general good agronomic and tillage practices and the planting of tolerant maize hybrids all form part of an integrated approach to reducing the probability of disease. Cultural methods are the main management strategies for S. maydis, since the fungus overwinters as pycnidia on corn residues (which can include leaves, stalks, and cobs) and can remain viable for at least one growing season. Seed treatments are fairly effective in controlling seedling blight, but once the fungus is established in the soil, crop rotation for at least two seasons is necessary to eliminate it.
Phytosanitary risk
Maize is an important silage and grain crop in the EPPO region, and S. maydis is one of the most prevalent ear and stalk rot pathogens of maize globally, causing reductions of grain quality and yield. There is the potential for further establishment in EPPO countries where it does not occur. Host plant availability and climate are favourable for the establishment of the fungi in maize-growing areas. There is the potential for further spread of this fungi in the EPPO region It has been repeatedly found in certain countries in the EPPO region, without having established.
PHYTOSANITARY MEASURES 2023-11-30
Certified Z. mays seed should be used. Seeds of Z. mays from countries where S. maydis occurs should come from pest free areas or in a pest-free place of production. For the inspection of consignments of seed or grain, the EPPO Standard PM 3/78(2) can be used (EPPO, 2021).
REFERENCES 2023-11-30
Baer OT, Laude TP, Reano CE, Gregorio GB, Diaz MGQ, Pabro LJA, Tamba L, Baltazar N, Fabreag MER, Pocsedio AE & Kumar AG (2021) Diplodia ear rot resistance QTL identified in maize (Zea mays l.) using multi-parent double-haploid population mapping. Sabrao Journal of Breeding and Genetics 53(1), 112–125.
Barros E, Crampton M, Marais G & Lezar S (2008) A DNA-based method to quantify Stenocarpella maydis in maize. Maydica 53(2), 125.
Chambers KR (1988) Effect of time of inoculation on Diplodia stalk and ear rot of maize in South Africa. Plant Disease 72, 529–531.
Christensen JJ & Wilcoxson RD (1967) Stalk rot of corn. Monograph of the American Phytopathological Society No. 3, 59 pp.
Dai K, Nagai M, Sasaki H, Nakamura H, Takechi K & Warabi M (1987) Detection of Diplodia maydis from imported corn seed. Research Bulletin of the Plant Protection Service of Japan 23, 1-6.
de la Riva A, García-Carneros AB & Molinero-Ruiz L (2019) First report of stalk rot of maize caused by Stenocarpella maydis in Spain. Plant Disease 103, 1789.
Dhanraj KS (1966) Dry rot of maize caused by Diplodia macrospora. Indian Phytopathology 19, 120.
EFSA PLH Panel (EFSA Panel on Plant Health), Bragard C, Baptista P, Chatzivassiliou E, Di Serio F, Gonthier P, Jaques Miret JA, Justesen AF, MacLeod A, Magnusson CS, Milonas P, Navas-Cortes JA, Parnell S, Potting R, Stefani E, Thulke H-H, Van der Werf W, Civera AV, Yuen J, Zappala L, Migheli Q, Vloutoglou I, Maiorano A, Streissl F & Reignault PL (2022). Scientific Opinion on the pest categorisation of Stenocarpella maydis. EFSA Journal 20(11), 7626, 29 pp. https://doi.org/10.2903/j.efsa.2022.7626
EPPO (2023) EPPO Global Database. https://gd.eppo.int/
EPPO (2021) PM 3/78 (2) Consignment inspection of seed and grain of cereals. EPPO Bulletin 51, 387-396.
Farr DF & Rossman AY (2022) Fungal Databases. National Fungus Collections, ARS, USDA, US. Available online: https://nt.ars-grin.gov/fungaldatabases/ [Accessed 10 September 2023].
Flett BC & Wehner FC (1991) Incidence of Stenocarpella and Fusarium cob rots in monoculture maize under different tillage systems. Journal of Phytopathology 133(4), 327–333.
Flett BC, Wehner FC & Smith MF (1992) Relationship between maize stubble placement in soil and survival of Stenocarpella maydis (Diplodia maydis). Journal of Phytopathology 134(1), 33–38.
Hooker AL & Britton MP (1962) The effects of stalk rot on corn yields in Illinois. Plant Disease 72, 529–531.
Koehler B (1960) Corn stalk rots in Illinois. Bulletin, University of Illinois Agricultural Experiment Station No. 658.
Luna MPR, Cambareto JJ & Wise KA (2017) Survival of Stenocarpella maydis on corn residue in Indiana. Plant Health Progress 18(2), 78–83.
McNew GL (1937) Crown infection of corn by Diplodia zeae. Iowa Agriculture Experimental Station Research Bulletin 19, 189–222.
Masango MG, Flett BC, Ellis CE & Botha CJ (2015) Stenocarpella maydis and its toxic metabolites: A South African perspective on diplodiosis. World Mycotoxin Journal 8, 341–350.
Nwigwe C (1974) Effect of Diplodia zeae and B-Phomopsis on the germination of seeds of maize (Zea mays). Plant Disease Reporter 58, 414-415.
Pinto FAMF, Porto VBC, Guimarães RA, Siqueira CDS, Faria MRD, Machado JDC & Medeiros FHVD (2022) Detection and factors that induce Stenocarpella spp. survival in maize stubble and soil suppressiveness under tropical conditions. Applied Sciences 12(10), 4974.
Shurtleff MC (1980) A Compendium of Corn Diseases, pp. 27, 44-45, 51-52. American Phytopathological Society, St Paul, Minnesota, USA.
Snyman LD, Kellerman TS, Vleggaar R, Flett BC, Basson KM & Schultz RA (2011) Diplonine, a neurotoxin isolated from cultures of the fungus Stenocarpella maydis (Berk.) Sacc. that induces diplodiosis. Journal of Agriculture and Food Chemistry 59, 9039-9044.
Steyn PS, Wessels PL, Holzapfel CW, Potgieter DJJ & Louw WKA (1972) The isolation and structure of a toxic metabolite from Diplodia maydis (Berk.) Sacc. Tetrahedron 28, 4775-4785.
Sutton BC & Waterston JM (1966) Diplodia maydis. CMI Descriptions of Pathogenic Fungi and Bacteria No. 84. CAB International, Wallingford, UK.
Walker JC (1969) Plant Pathology (3rd edition), pp. 335, 341. McGraw-Hill, New York, USA.
Wicklow DT, Rogers KD, Dowd PF & Gloer JB (2011) Bioactive metabolites from Stenocarpella maydis, a stalk and ear rot pathogen of maize. Fungal Biology 115, 133-142.
Xia Z & Achar PN (2001) Random amplified polymorphic DNA and polymerase chain reaction markers for the differentiation and detection of Stenocarpella maydis in maize seeds. Journal of Phytopathology 149(1), 35-44.
Zad J & Ale Agha N (1985) A note on the mycoflora of maize in Iran. Mededelingen van de Faculteit Landbouwwetenschappen Rijksuniversiteit Gent 50, 1149-1151.
ACKNOWLEDGEMENTS 2023-11-30
This datasheet was extensively revised in 2023 by Felipe A. M. Ferreira Pinto, Epagri Santa Catarina- Brazil. His valuable contribution is gratefully acknowledged.
How to cite this datasheet?
Datasheet history 2023-11-30
This datasheet was first published in the EPPO Bulletin in 1982 and revised in the two editions of 'Quarantine Pests for Europe' in 1992 and 1997, as well as in 2023. It is now maintained in an electronic format in the EPPO Global Database. The sections on 'Identity', ‘Hosts’, and 'Geographical distribution' are automatically updated from the database. For other sections, the date of last revision is indicated on the right.
CABI/EPPO (1992/1997) Quarantine Pests for Europe (1st and 2nd edition). CABI, Wallingford (GB).
EPPO (1982) Data sheets on quarantine organisms, Diplodia maydis. EPPO Bulletin 12(1), 49-53. https://onlinelibrary.wiley.com/doi/epdf/10.1111/j.1365-2338.1982.tb01955.x
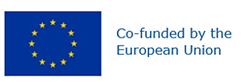