Homalodisca vitripennis(HOMLTR)
EPPO Datasheet: Homalodisca vitripennis
Homalodisca vitripennis is a highly polyphagous pest that vectors Xylella fastidiosa.
IDENTITY
Authority: (Germar)
Taxonomic position: Animalia: Arthropoda: Hexapoda: Insecta: Hemiptera: Auchenorrhyncha: Cicadellidae
Other scientific names: Homalodisca coagulata (Say), Homalodisca triquetra auctorum, Tettigonia coagulata Say, Tettigonia vitripennis Germar
Common names in English: glassy-winged sharpshooter
view more common names online...
Notes on taxonomy and nomenclature
The taxonomic revision that synonymized Homalodisca coagulata with H. vitripennis is detailed in Takiya et al. (2006). H. coagulata was used in the scientific literature from 1958 following Young’s reinstatement of H. coagulata as the valid name for the glassy-winged sharpshooter. Prior to the revision of Takiya et al. (2006) a considerable body of work on the ecology, management, and biology of the glassy-winged sharpshooter, published in the 1990s and early 2000s, refer to this pest as H. coagulata. As such, literature reviews on the glassy-winged sharpshooter should be conducted using Homalodisca coagulata and Homalodisca vitripennis as key words. The common name, sharpshooter, refers to species in the tribes Cicadellini (cosmopolitan in distribution but species richness is greatest in the neotropics) and Proconiini (restricted to the New World) within the subfamily Cicadellinae, which is contained in the family Cicadellidae within the Hemiptera.
EU Categorization: A1 Quarantine pest (Annex II A)
view more categorizations online...
EPPO Code: HOMLTR
HOSTS 2021-03-08
Homalodisca vitripennis is xylophagous and this insect uses its needle-like mouthparts, specifically the maxillary stylets (Leopold et al., 2003), to penetrate plant material to reach the xylem from which fluids are ingested. Xylem fluids are nutritionally poor and carbohydrates occur in very low concentrations. Amino acids and organic acids are the dominant compounds found in xylem fluid (Andersen et al., 1992). Low quality food and xylem-fluid chemistry that fluctuates seasonally affect concentrations of essential nutrients. This, may in part, drive polyphagy and seasonal host use patterns exhibited by H. vitripennis (Andersen et al., 1992). Total amino acids, amides, and glutamine in xylem fluid and xylem tension are correlated with feeding rates (Andersen et al., 1992) which may influence choice of plants to feed on at different times of the year. These factors, especially xylem tension and the associated difficulty of extracting fluids, may have driven the evolution of large size in sharpshooters (e.g. Proconiini) as increasing body size mitigates energetic costs of feeding on this resource (Novotny and Wilson, 1997).
Consequently, H. vitripennis has been recorded feeding on more than 100 different hosts (Adlerz, 1980) in at least 37 different families (Hoddle et al., 2003; Rathe et al., 2012; Groenteman et al., 2015; Turner and Pollard, 1959) that span across grasses to plants having woody branches. However, it is not clear how many of these feeding hosts can support the complete development of H. vitripennis, and there may be a requirement for nymphs to feed on different host plant species over the course of their lifetime (Andersen et al., 1992; Sorensen and Gill, 1996). The host list of H. vitripennis includes some economically important plants (e.g. Citrus limon, C. sinensis, Glycine max, Helianthus annuus, Juglans regia, Malus spp. Medicago sativa, Olea europaea, Persea americana, Prunus domestica, P. dulcis, P. persica, Vaccinium spp., Vitis vinifera, Zea mays) and some common ornamental species (e.g. Camellia japonica, Hibiscus rosa-sinensis, Lagerstroemia indica, Nerium oleander).
The known host lists that are available may not include plant species that H. vitripennis can feed and reproduce on should it invade and establish in new areas. There are three possible ways to proactively determine which novel host plant species H. vitripennis could potentially be exposed to and feed on should it invade a new region. Rathe et al. (2014) conducted a two-year garden experiment in California (USA), an area with invasive H. vitripennis populations, to assess the preference of this pest for native Australian plants that may be at risk of attack should H. vitripennis establish in Australia. Groenteman et al. (2015) surveyed botanic gardens, arboreta, and landscape plantings in commercial, recreational, and urban areas in California for evidence of H. vitripennis associations with native New Zealand plants. Both New Zealand and Australia are concerned about the biosecurity risks this pest poses to horticultural industries and native plants. Studies similar to those conducted by Rathe et al. (2014) and Groenteman et al. (2015) could also be run within the native range of H. vitripennis (i.e. the South-Eastern USA) instead of invaded areas (i.e. California). An alternative approach to field surveys to gauge risk to novel plant species from H. vitripennis is to conduct ecological niche modelling (e.g. CLIMEX) that assesses establishment likelihoods based on climate suitability for H. vitripennis populations (Hoddle, 2004; Rathe et al., 2012; Charles and Logan, 2013) and degree-days required for development (Pilkington et al., 2014). Predictive maps from models may provide insight into possible novel host plant species H. vitripennis would be exposed to should successful incursion, establishment, and spread occur in previously uncolonized areas.
Host list: Abelmoschus esculentus, Abrus precatorius, Acer rubrum, Albizia julibrissin, Alcea rosea, Amaranthus hybridus, Amaranthus spinosus, Amaranthus viridis, Ambrosia artemisiifolia, Ampelopsis arborea, Asclepias sp., Asparagus officinalis, Baccharis halimifolia, Begonia sp., Bidens pilosa, Bucida buceras, Caesalpinia pulcherrima, Callicarpa americana, Camellia japonica, Campsis radicans, Carphephorus odoratissimus, Carya illinoinensis, Cassia sp., Casuarina, Catalpa bignonioides, Catalpa sp., Cenchrus purpureus, Cephalanthus occidentalis, Cercis sp., Chenopodium album, Chrysanthemum x morifolium, Citrus sp., Citrus x aurantium var. sinensis, Citrus x limon, Clerodendrum indicum, Clusia sp., Codiaeum variegatum, Conocarpus erectus, Convolvulus arvensis, Cordyline fruticosa, Cotoneaster sp., Cycas sp., Dysphania ambrosioides, Elaeagnus sp., Enterolobium cyclocarpum, Erechtites hieraciifolius, Erigeron canadensis, Eriobotrya japonica, Eucalyptus sp., Eupatorium capillifolium, Eupatorium perfoliatum, Ficus benjamina, Ficus sp., Fortunella sp., Fraxinus sp., Gardenia sp., Gardenia taitensis, Ginkgo biloba, Gladiolus sp., Glycine max, Gordonia lasianthus, Gossypium herbaceum, Helianthus annuus, Helianthus, Hemionitis arifolia, Heptapleurum actinophyllum, Heterotheca subaxillaris, Hibiscus rosa-sinensis, Ilex glabra, Ilex vomitoria, Ilex, Indigofera hirsuta, Ipomoea, Jasminum mesnyi, Juglans regia, Lactuca canadensis, Lagerstroemia indica, Lagerstroemia speciosa, Lantana camara, Leucadendron sp., Ligustrum sp., Liquidambar styraciflua, Ludwigia peruviana, Macadamia sp., Magnolia virginiana, Malus sylvestris, Mangifera indica, Manilkara roxburghiana, Medicago sativa, Melaleuca quinquenervia, Melaleuca viminalis, Melia azedarach, Mimosa sp., Mirabilis jalapa, Momordica charantia, Monarda fistulosa, Morella cerifera, Myrtus communis, Nerium oleander, Nyssa sylvatica, Oenothera laciniata, Oenothera simulans, Olea europaea, Olea sp., Ostrya virginiana, Parkinsonia aculeata, Parthenocissus quinquefolia, Persea americana, Photinia sp., Phytolacca americana, Pinus sp., Pisum sp., Pittosporum sp., Platanus sp., Populus sp., Prunus angustifolia, Prunus caroliniana, Prunus domestica, Prunus dulcis, Prunus persica, Prunus serotina, Prunus sp., Psidium, Psophocarpus tetragonolobus, Pteridium aquilinum, Pyracantha coccinea, Pyracantha sp., Pyrus communis, Pyrus sp., Quercus laevis, Quercus sp., Quercus virginiana, Quercus, Rhus sp., Ricinus communis, Rosa sp., Rubus sp., Rudbeckia laciniata, Salix caroliniana, Salix, Sambucus canadensis, Sansevieria sp., Sassafras albidum, Schinus terebinthifolia, Senna occidentalis, Senna tora, Sida cordifolia, Sida rhombifolia, Smilax laurifolia, Solidago altissima, Solidago fistulosa, Solidago sp., Sonchus asper, Sorghum bicolor, Sorghum halepense, Spathodea campanulata, Swietenia mahagoni, Tetragonolobus sp., Thuja sp., Urena lobata, Vernicia fordii, Viburnum sp., Vigna unguiculata, Vitis munsoniana, Vitis vinifera, Vitis, Wisteria sp., Xanthium sp., Yucca aloifolia, Zea mays, x Citrofortunella microcarpaGEOGRAPHICAL DISTRIBUTION 2021-03-08
Homalodisca vitripennis is native to the South-Eastern USA and North-Eastern Mexico (Triapitsyn and Phillips, 2000). Within this native range there is high variability in H. vitripennis abundance, with it being more common in Eastern Texas, Georgia, and Northern Florida, and less common in Central and South Florida and North-Eastern Mexico (e.g. Tamaulipas) (Triapitsyn and Phillips, 2000). Sometime in the 1980s, H. vitripennis was accidentally introduced into Southern California (USA), most likely through the trade in live ornamental plants that originated from the South-East USA (Blua et al., 1999; Sorensen and Gill, 1996). This pest has exhibited high invasion potential since its introduction in California. In 1999, H. vitripennis established in Tahiti and rapidly spread through French Polynesia (Grandgirard et al., 2006). In 2004, H. vitripennis was recorded from Hawaii, and then from Easter Island and the Cook Islands in 2005 and 2007, respectively (Petit et al., 2008; Rathe et al., 2015). Movement of live plants was the most likely conduit for long distance movement into new areas of the South Pacific. H. vitripennis egg masses on the undersides of leaves were probably the life stage that were moved undetected into new areas (Petit et al., 2009). Long distance movement of adult H. vitripennis in the absence of host plant material via aircraft may also be possible, but the fitness of surviving females may be compromised as a result of lack of food and low temperature exposure in cargo storage areas (Rathe et al., 2015).
South America: Chile (Easter Island)
Oceania: Cook Islands, French Polynesia
BIOLOGY 2021-03-08
Feeding Biology
H. vitripennis is a specialist xylem feeder and as a result of this xylophagy this pest exhibits an extremely high rate of feeding on nutritionally poor foods. Adult H. vitripennis can ingest up to 100 – 300 times their dry body weight in xylem fluid per day, and the larger-sized females tend to feed more than males (Brodbeck et al., 1993; Redak et al., 2004). Xylem fluids are nutritionally poor, however, extraction of amino acids, organic acids, and sugars ingested by sharpshooters, including H. vitripennis, is very efficient, approaching 99% (Andersen et al., 1989), and resulting excreta, which is copious, is primarily water, and is referred to as sharpshooter ‘rain’ (Blua et al., 1999). Sharpshooters engage in labial dabbing as they explore plant surfaces for feeding sites. Should a suitable feeding site be found, stylets are inserted intracellularly, in a nearly straight line, which allows rapid, sometimes within 1-2 minutes on suitable hosts, location of the xylem (Krugner et al., 2019). While probing, sharpshooters release two types of saliva, one type hardens and forms the salivary sheath that encloses the stylets, and the second is a digestive saliva that contains enzymes which loosen the xylem wall allowing access to internal xylem fluids (Krugner et al., 2019).
Host Plant Selection
Movement of H. vitripennis in the environment is driven by insect behaviour and host plant quality which varies temporally due to fluctuating xylem chemistry. H. vitripennis may use visual cues to initiate landing behaviours, and the presence of conspecifics on host plants may be one such stimulus (Mizell et al., 2012). Host plant quality affects attraction and retention rates with high quality host plants increasing patch residence times for H. vitripennis (Northfield et al., 2009). Dispersal capacity and host plant selection has been assessed in the field using mark-recapture techniques. H. vitripennis can move about 100-150 m over a 24-hour period (Krugner et al., 2019) and insects may aggregate on preferred host plants. Aggregation may be a response to plant species and vigour, which, with respect to peach trees in the South-East USA, trees in decline appear to induce aggregation by H. vitripennis (Krugner et al., 2019). In California, overwintering H. vitripennis populations tend to be greatest in citrus. These overwintering aggregations in citrus pose a significant threat to vineyards that are in close proximity to citrus orchards as H. vitripennis migrate from overwintering sites into grape production areas and this movement is correlated with outbreaks of Pierce’s disease, a lethal disorder of grapes caused by a xylem-dwelling bacterium, Xylella fastidiosa, that H. vitripennis vectors (see section on Pest Significance below). Irvin and Hoddle (2004) speculate that host plant oviposition preferences by H. vitripennis, particularly with respect to lemons, may be affected by leaf thickness and leaf surface topography.
Reproductive Behaviour and Biology
Sharpshooters lack pheromones for long range attraction (Krugner et al., 2019) and detection of conspecifics for mating occurs through acoustic communication via vibrational signals that are transmitted through host plant substrates (Nieri et al., 2017). For vibrational communication to be effective, conspecifics need to be on the same plant, or if on separate plants, the plants need to be in contact for signal transmission to occur. Vibrational communications tend to be initiated by female H. vitripennis and males respond which results in a communication duet of female-male calls (Nieri et al., 2017). Physical courtship behaviour between males and females takes on average about 6 mins to complete and copulation lasts for about 165 mins (Hix, 2001). Females are receptive to mating 96 hrs post-eclosion, however, some females may not copulate until 14 days post-eclosion. Females mate once and males tend to mate multiple times (Hix, 2001). Following mating, females engage in a variety of behaviours that result in the deposition of a chalky white material, brochosomes, being excreted from the anus, which is then prepared through kneading with tarsi for deposition on the hindwings where it appears as two distinct white spots (Hix, 2001). Following brochosome deposition on wings, females commence searching for suitable oviposition sites. To oviposit eggs, females use the ovipositor to make a slit in the epidermis on the underside of the leaf into which the ovipositor is inserted. Eggs tend to be deposited sequentially in pairs (Hix, 2001) under the epidermis next to the parenchyma (Irvin and Hoddle, 2004) and the oviposition slit in the epidermis is enlarged to accommodate additional eggs (Hix, 2001). Females, on average, oviposit about 12-13 eggs per egg mass (for odd numbers of eggs, a single egg is always deposited last), a process which takes about 48 mins, on average, to complete (Hix, 2001). Each time eggs are laid, the female removes brochosomes from the wings, and this white powder is used to cover the newly oviposited eggs (Hix, 2001). Several functions have been proposed for brochosomes covering sharpshooter egg masses. These potential uses include protection of eggs from desiccation, UV light, egg parasitoids and predators, and entomopathogenic fungi, and possibly to advertise to other females that suitable oviposition sites have already been used (Hix, 2001). It has been demonstrated experimentally that brochosomes are a hindrance to parasitoids that attack H. vitripennis eggs (Velema et al., 2005). Interestingly, unmated females do not secrete brochosomes (Hix, 2001). Hummel et al. (2006a,b,c) describe female H. vitripennis genitalia, reproductive organs, and seasonal patterns of reproductive physiology that can be assessed to determine the probable number of H. vitripennis generations per year. In the laboratory at 25oC, reproductively active, females are capable of laying an average of around 214 eggs over their lifetime (Pilkington et al., 2014). H. vitripennis overwinter as reproductively inactive adults (Hummel et al., 2006c).
Developmental Biology
H. vitripennis has seven life stages: eggs, five nymphal instars, and adults (males and females). Development times, fecundity, longevity, and stage specific survivorship rates are strongly affected by temperature (Pilkington et al., 2014). The degree-day requirements for H. vitripennis development together with estimates of upper, lower, and optimal temperature limits have been determined in the laboratory across a range of constant temperatures (Pilkington et al., 2014). These data have been used to calculate average time in days for developmental stages, stage-specific survivorship rates, adult longevity, and fecundity. These data, in part, allowed the calculation of key life table parameters (e.g. net reproductive rate, Ro) for H. vitripennis under constant temperatures in the laboratory (Pilkington et al., 2014; Sétamou and Jones, 2005). Practical application of these data enables estimation of the number of generations H. vitripennis is likely to have in a given area, understanding incursion risk and establishment of year-round populations, and for optimizing laboratory rearing conditions (Pilkington et al., 2014; Sétamou and Jones, 2005).
DETECTION AND IDENTIFICATION 2021-03-08
Symptoms
Sharpshooter ‘rain’ is excreta composed almost entirely of water that is excreted by feeding nymphs and adults. When H. vitripennis populations are heavy, ‘rain’ is easily seen and readily felt landing on skin and hair when standing under infested trees. Video of H. vitripennis rain in Tahiti is available here. Copious amounts of excreta can dry on leaves and fruit which results in a chalky white powdery appearance. Adult and nymphal sharpshooters are large and easily visible but tend to move in a quick shuffling like motion around twigs or branches to hide when being observed. Adults readily fly if disturbed unexpectedly and they are attracted to lights at night. High densities of feeding sharpshooters may reduce plant vigour and adversely affect fruit production.
Morphology
Eggs
Female H. vitripennis lay eggs on the underside of leaves. The ovipositor is used to make a slit in the epidermis and eggs are deposited underneath the epidermis. Eggs are approximately 2mm in length (Hummel et al., 2006b), and are laid in batches of around 12-13 eggs (Hix, 2001). Eggs laid in a batch are collectively referred to as an egg mass.
Nymphs
H. vitripennis has five nymphal instars and undergo four moults to reach the fifth instar. Nymphs tend to be greyish with reddish eyes. Nymphs are flightless and wing pads enlarge after each successive moult. Sétamou and Jones (2005) provide measurements for head capsule widths, hind tibia and body lengths for nymphs.
Adults
Adults are mottled brown/black in coloration and approximately 1cm in length (Sétamou and Jones, 2005). Young adults tend to have reddish wings which darken as they age, and this phenomenon may be useful for estimating adult age (Timmons et al., 2011). Mated females anoint their hindwings with white brochosomes and these appear as vivid white dots (Hix, 2001).
Detection and inspection methods
Egg masses, nymphs and adults can be detected via visual inspections of plants. However, this is time-consuming and movement of foliage will disturb nymphs and adults causing them to abandon plants. Egg masses covered with white brochosomes are easier to detect visually than egg masses that lack brochosomes. Adult H. vitripennis are attracted to yellow sticky cards and cards are an excellent passive method of detection. Sweep netting of foliage will capture adults and nymphs.
PATHWAYS FOR MOVEMENT 2021-03-08
The highest risk pathway for movement of H. vitripennis eggs, nymphs, and adults is the movement of live plants which has almost certainly resulted in the accidental translocation of H. vitripennis over vast distances into new areas. Adults may be moved to new areas inside of aircraft, but this is probably a lower risk pathway when compared to live plant movement (Rathe et al., 2015).
PEST SIGNIFICANCE 2021-03-08
Economic impact
The most significant economic problem associated with H. vitripennis is the vectoring of xylem-dwelling bacteria that cause scorch-like diseases in economically important crops (e.g. grapes) and ornamental plants (e.g. oleander). A notorious example is Xylella fastidiosa, the causative agent of Pierce’s Disease of grapes, which is vectored by H. vitripennis.
With respect to Pierce’s Disease in California, H. vitripennis increased disease severity because high density populations increased vectoring capacity. Its natural dispersal capabilities are substantially greater than smaller native sharpshooter species (e.g., Graphocephala atropunctata), it exploits a diverse array of native and exotic plants for feeding and reproduction than native vectors, and adult H. vitripennis have been demonstrated to engage in plant-to-plant spread of X. fastidiosa that results in rapid bacterial spread and disease outbreaks (CDFA, 2019).
In response to the threat the H. vitripennis-X. fastidiosa combination poses, the California Department of Food and Agriculture (CDFA) has implemented a rigorous management program to contain the spread of H. vitripennis that could be accidentally moved on ornamental and nursery stock plants that originate in Southern California and are shipped to Northern California where wine grape production is concentrated (CDFA, 2019). This program has been ongoing for more than 20 years and is comprised of five major components: (1) containing spread of H. vitripennis from infested areas into non-infested regions, (2) statewide survey and detection programs for incursions, (3) rapid response reactions to confirmed detections (18 incipient H. vitripennis populations have been eradicated as part of this program), (4) research into H. vitripennis and X. fastidiosa management (34.3 million USD has been used to fund 225 research projects), and (5) environmental compliance reviews to ensure that management activities are conducted in an environmentally responsible manner (CDFA, 2019).
This high level of investment has been needed because of the enormous economic value of crops that are at risk from strains of X. fastidiosa that H. vitripennis is capable of vectoring. With respect to grapes in California, Pierce’s disease threatens a crop with production value of 6.25 billion USD and associated economic activity (e.g. vineyard tours and associated wine-related tourism) of approximately 57.6 billion USD. Other crops such as almonds (5.47 billion USD) and citrus (1.12 billion USD), stone fruits (885 million USD), and ornamental shade trees are also at risk, either from the strains of X. fastidiosa found in California, or from related strains found elsewhere in the world that could accidentally enter, establish, and be spread by H. vitripennis in California (e.g. citrus variegated chlorosis caused by X. fastidiosa subsp. pauca (CDFA, 2019). Economic forecasting studies suggest that failure to contain and control H. vitripennis would cost the grape industry more than 180 million USD annually (Alston et al., 2013). Additionally, costs to industries, such as producers of ornamental plants, to mitigate H. vitripennis movement and to meet quarantine regulations for shipping plants, have been substantial (Jetter et al., 2014).
In French Polynesia, high density uncontrolled H. vitripennis populations caused significant social and environmental problems. At night adult sharpshooters invaded houses in the hundreds by flying through open windows in response to being attracted to lights. A regular morning routine was the sweeping up and removal of dead adults littering floors and verandas. Other annoyances were caused by adult sharpshooters landing on exposed skin and ‘biting’ people as sweat glands were probed and auditory irritation caused by flying adults ‘buzzing’ around ears and heads, especially at night when home invasions occurred. Production (quality and quantity) of some fruit species (e.g., mangoes) reportedly declined due to large feeding populations of H. vitripennis. These purported impacts on fruit production were not quantified.
Alarmingly, H. vitripennis was documented as having a negative impact on native spider populations in French Polynesia. Lab and field studies indicated that H. vitripennis adults and nymphs were toxic to some species of spider that consumed them. The mechanism underlying this putative toxic effect is unknown (Suttle and Hoddle, 2006).
Control
A variety of control options are available for managing H. vitripennis. Insecticides are effective, especially low rates of systemic insecticides. However, excessive use over long periods of time results in resistance development (Andreason et al., 2018). Cultural control approaches have investigated the use of barriers to reduce immigration rates of H. vitripennis into vineyards (Blua et al., 2005). Attempts at behavioural control have used vibrational interference to reduce the likelihood of mating success in vineyards (Krugner and Gordon, 2018). Significant permanent control of high density invasive H. vitripennis populations has been rapidly achieved through importation (= classical) biological with the mymarid egg parasitoid Cosmocomoidea ashmeadi (Girault) (formerly Gonatocerus ashmeadi Girault) (Grandgirard et al., 2008). This natural enemy is a key component that is necessary for sustainable control of H. vitripennis.
Phytosanitary risk
H. vitripennis is a significant phytosanitary risk primarily because it vectors xylem-dwelling bacteria, such as Xylella fastidiosa, that have the potential to cause significant scorch-like plant diseases (e.g. Pierce’s Disease of grapes, almond leaf scorch, oleander leaf scorch, and diseases of olives). As X. fastidiosa outbreaks have been detected in the EPPO region, the possible introduction of a polyphagous and effective vector such as H. vitripennis would further complicate disease management.
PHYTOSANITARY MEASURES 2021-03-08
Phytosanitary measures to mitigate invasion risk need to revolve around the managing hazards associated with the trade and exchange of live plants which have the potential to move H. vitripennis from areas where it is established into regions where it is not currently present. If not already covered by specific phytosanitary requirements, it can be generally recommended that plants to be used in planting programs and are known hosts of H. vitripennis should come from areas that are free of the pest, or that have been produced and transported under conditions that significantly reduce the likelihood of accidental pest infestation.
REFERENCES 2021-03-08
Adlerz WC (1980) Ecological observations on two leafhoppers that transmit the Pierce’s disease bacteria. Proceedings of the Florida State Horticultural Society 93, 115-120.
Alston JM, Fuller KB, Kaplan JD, & Tumber KP (2013) The economic consequences of Pierce’s Disease and related policy in the California wine grape industry. Journal of Agricultural and Resource Economics 38(2), 269-297
Andersen PC, Brodbeck BV & Mizell III RF (1989) Metabolism of amino acids, organic acids and sugars extracted from the xylem fluid of four host plants by adult Homalodisca coagulata. Entomologia Experimentalis et Applicata 50,149–159.
Andersen PC, Broadbeck BV & Mizell III RF (1992) Feeding by the leaf hopper, Homalodisca coagulata, in relation to xylem chemistry and tension. Journal of Insect Physiology 38(8), 611-622.
Andreason SA, Prabhaker N, Castle SJ, Ganjisaffar F, Haviland DR, Stone-Smith B & Perring TM (2018) Reduced susceptibility of Homalodisca vitripennis (Hemiptera: Cicadellidae) to commonly applied insecticides. Journal of Economic Entomology 111(5),
Blua MJ, Phillips PA & Redak RA (1999) A new sharpshooter threatens both crops and ornamentals. California Agriculture 53(2), 22-25.
Blua MJ, Campbell K, Morgan DJW & Redak RA (2005) Impact of a screen barrier on dispersion behavior of Homalodisca coagulata (Hemiptera: Cicadellidae). Journal of Economic Entomology 98(5),
Brodbeck BV, Mizell III RF, French WJ, Andersen PC & Aldrich JH (1993) Physiological and behavioral adaptations of three species of leafhoppers in response to the dilute nutrient content of xylem fluid. Journal of Insect Physiology 39, 73–81.
California Department of Food and Agriculture (CDFA) (2019) Pierce’s Disease Control Program Report to the Legislature for calendar year 2019. https://www.cdfa.ca.gov/pdcp/Documents/LegReport/2019LegReport.pdf (last accessed 18 Feb. 2021).
Charles JG & Logan DP (2013) Predicting the distribution of Gonatocerus ashmeadi, an egg parasitoid of glassy winged sharpshooter, in New Zealand. New Zealand Entomologist 36(2), 73-81.
Grandgirard J, Hoddle MS, Roderick GK, Petit JN, Percy D, Putoa R, Garnier C & Davies N (2000) Invasion of French Polynesia by the glassy-winged sharpshooter, Homalodisca coagulata (Hemiptera: Cicadellidae): A new threat to the South Pacific. Pacific Science 60(4), 429-438.
Grandgirard J, Hoddle MS, Petit JN, Roderick GK & Davies N (2008) Engineering an invasion: classical biological control of the glassy-winged sharpshooter, Homalodisca vitripennis, by the egg parasitoid Gonatocerus ashmeadi in Tahiti and Moorea, French Polynesia. Biological Invasions 10, 135-148.
Groenteman R, Forgie SA, Hoddle MS, Ward DF, Goeke DF & Anand N (2015) Assessing invasion threats: novel insect-pathogen-natural enemy associations with native New Zealand plants in southern California. Biological Invasions 17,
Hix RL (2001) Egg-laying and brochosome production observed in glassy-winged sharpshooter. California Agriculture 55(4), 19-22.
Hoddle MS, Triaptisyn SV & Morgan DJW (2003) Distribution and plant association records for Homalodisca coagulata (Hemiptera: Cicadellidae) in Florida. Florida Entomologist 86(1), 89-91.
Hoddle MS (2004) The potential adventive geographic range of glassy-winged sharpshooter, Homalodisca coagulata and the grape pathogen Xylella fastidiosa: implications for California and other grape growing regions of the world. Crop Protection 23, 691-699.
Irvin NA & Hoddle MS (2004) Oviposition preference of Homalodisca coagulata for two Citrus limon cultivars and influence of host plant on parasitism by Gonatocerus ashmeadi and G. triguttatus (Hymenoptera: Mymaridae). Florida Entomologist 87, 504-510.
Hummel NA, Zalom FG & Peng CYS (2006a) Structure of female genitalia of glassy-winged sharpshooter, Homalodisca coagulata (Say) (Hemiptera: Cicadellidae). Arthropod Structure & Development 35, 111-125.
Hummel NA, Zalom FG & Peng CYS (2006b) Anatomy and histology of reproductive organs of female Homalodisca coagulata (Hemiptera: Cicadellidae: Proconiini), with special emphasis on categorization of vitellogenic oocytes. Annals of the Entomological Society of America 99, 920-932.
Hummel NA, Zalom FG, Toscano NC, Burman P & Peng CYS (2006c) Seasonal patterns of female Homalodisca coagulata (Say) (Hemiptera: Cicadellidae) reproductive physiology in Riverside, California. Environmental Entomology 35, 901-906.
Jetter KM, Morse JG & Kabashima JN (2014) The cost of the glassy-winged sharpshooter to California grape, citrus and nursery producers. California Agriculture 68(4), 161-167.
Krugner R & Gordon SD (2018) Mating disruption of Homalodisca vitripennis (Germar) (Hemiptera: Cicadellidae) by playback of vibrational signals in vineyard trellis. Pest Management Science 74,
Krugner R, Sistertson MS, Backus EA, Burbank LP & Redak RA (2019) Sharpshooters: A review of what moves Xylella fastidiosa. Austral Entomology 58, 248-267.
Leopold RA, Freeman TP, Buckner JS & Nelson DR (2003) Mouthpart morphology and stylet penetration of host plants by the glassy-winged sharpshooter, Homalodisca coagulata, (Hemiptera: Cicadellidae). Arthropod Structure & Development 32, 189-199.
Mizell RF, Andersen PC, Brodbeck BV & Hunter WB (2012) Congener response reduces risks from bottom-up and top-down forces: behavioral parsimony by a xylophage. American Entomologist 58, 106–115.
Nieri R, Mazzoni V, Gordon SD & Krugner R (2017) Mating behavior and vibrational mimicry in the glassy-winged sharpshooter, Homalodisca vitripennis. Journal of Pest Science 90, 887–899.
Northfield TD, Mizell III RF, Paini DR, Andersen PC, Brodbeck BV, Riddle TC & Hunter WB (2009) Dispersal, patch leaving, and distribution of Homalodisca vitripennis (Hemiptera: Cicadellidae). Environmental Entomology 38, 183–191.
Novotny V & Wilson MR (1997) Why are there no small species among xylem-sucking insects? Evolutionary Ecology 11, 419-437.
Petit JN, Hoddle MS, Grandigirard J, Roderick GK & Davies N (2008) Invasion dynamics of the glassy-winged sharpshooter Homalodisca vitripennis (Germar) (Hemiptera: Cicadellidae) in French Polynesia. Biological Invasions 10, 955-967.
Petit JN, Hoddle MS Grandigirard J, Roderick GK & Davies N (2009) Successful spread of a biocontrol agent reveals a biosecurity failure: elucidating long distance invasion pathways for Gonatocerus ashmeadi in French Polynesia. BioControl 54, 485-495.
Pilkington LJ, Lewis M, Jeske D & Hoddle MS (2014) Calculation and thematic mapping of demographic parameters for Homalodisca vitripennis (Hemiptera: Cicadellidae) in California. Annals of the Entomological Society of America 107(2), 424-434.
Rathe AA, Pilkington LJ, Gurr GM, Hoddle MS, Daugherty MP, Constable FE, Luck JE, Powell KS, Fletcher MJ & Edwards OR (2012) Incursion preparedness: anticipating the arrival of an economically important plant pathogen Xylella fastidiosa Wells Proteobacteria: Xanthomonadaceae) and the insect vector Homalodisca vitripennis (Germar) (Hemiptera: Cicadellidae) in Australia. Australian Journal of Entomology 51, 209-220.
Rathe AA, Pilkington LJ, Hoddle MS, Spohr LJ, Daugherty MP & Gurr GM (2014) Feeding and development of the glassy-winged sharpshooter, Homalodisca vitripennis, on Australian native plant species and implications for Australian biosecurity. PLoS 1 9(3): e90410.
Rathe AA, Pilkington LJ, Spohr LJ, Hoddle MS, Daugherty MP & Gurr GM (2015) Invasion pathway risk analysis for the glassy-winged sharpshooter (Homalodisca vitripennis): survival and reproductive success following simulated air transportation. Biological Invasions 17,
Redak RA, Purcell AH, Lopes JRS, Blua MJ, Mizell III RF & Andersen PC (2004) The biology of xylem-feeding insect vectors of Xylella fastidiosa and their relation to disease epidemiology. Annual Review of Entomology 49, 243-270.
Sétamou M & Jones WA (2005) Biology and biometry of Homalodisca coagulata (Homoptera: Cicadellidae) reared on cowpea. Annals of the Entomological Society of America 98, 322-328.
Sorensen JT & Gill RJ (1996) A range extension of Homalodisca coagulata (Say) (Hemiptera: Clypeorrhyncha: Cicadellidae) to Southern California. Pan-Pacific Entomologist 72, 160-161.
Suttle BK & Hoddle MS (2006) Engineering enemy-free space: An invasive pest that kills its predators. Biological Invasions 8(4), 639-649.
Takiya DM, McKamey SH & Cavichioli RR (2006) Validity of Homalodisca and H. vitripennis as the name for glassy-winged sharpshooter (Hemiptera: Cicadellidae: Cicadellinae). Annals of the Entomological Society of America 99(4), 648-655.
Timmons C, Hassell A, Lauziere I & Bextine B (2011) Age determination of the glassy-winged sharpshooter, Homalodisca vitripennis, using wing pigmentation. Journal of Insect Science 11(78), 1-9.
Triapitsyn SV & Phillips PA (2000) First record of Gonatocerus triguttatus (Hymenoptera: Mymaridae) from eggs of Homalodisca coagulata (Homoptera: Cicadellidae) with notes on the distribution of the host. Florida Entomologist 82, 200-203.
Turner WF & Pollard HN (1959) Life histories and behavior of five insect vectors of phony peach disease. USDA Technical Bulletin 1188, 28 pp.
Velema HP, Hemerik L, Hoddle MS & Luck RF (2005) Brochosome influence on parasitisation efficiency of Homalodisca coagulata (Say) (Hemiptera: Cicadellidae) egg masses by Gonatocerus ashmeadi Girault (Hymenoptera: Mymaridae). Ecological Entomology 30, 485-496.
Young DA (1958) A synopsis of the species of Homalodisca in the United States (Homoptera: Cicadellidae). Bulletin of the Brooklyn Entomological Society 53, 7-13.
ACKNOWLEDGEMENTS 2021-03-08
This datasheet was prepared in 2021 by Mark Hoddle, University of California, Riverside. His valuable contribution is gratefully acknowledged.
How to cite this datasheet?
Datasheet history 2021-03-08
This datasheet was first published online in 2021. It is maintained in an electronic format in the EPPO Global Database. The sections on 'Identity', ‘Hosts’, and 'Geographical distribution' are automatically updated from the database. For other sections, the date of last revision is indicated on the right.
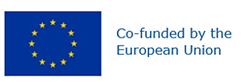