Emaravirus rosae(RRV000)
EPPO Datasheet: Emaravirus rosae
IDENTITY
Taxonomic position: Viruses and viroids: Riboviria: Orthornavirae: Negarnaviricota: Polyploviricotina: Bunyaviricetes: Elliovirales: Fimoviridae: Emaravirus
Other scientific names: RRV, rose rosette emaravirus, rose rosette virus
Common names in English: rose rosette disease
view more common names online...
Notes on taxonomy and nomenclature
The International Committee on Taxonomy of Viruses (ICTV) approved and ratified relevant changes to virus taxonomy in March 2021. The ICTV mandated a uniform rule for virus species naming, which follows the binomial ’genus-species’ format with or without Latinized species epithets, and all Study Groups were requested to convert all previously established species names to the new format. The ICTV has also abolished the notion of a type species, i.e., a species chosen to serve as a name-bearing type of a virus genus (Walker et al., 2021). From here, the preferred name for the disease is rose rosette disease, the virus name Emaravirus rosae, and the virus acronym RRV.
EU Categorization: Emergency measures (formerly), A1 Quarantine pest (Annex II A)
view more categorizations online...
EPPO Code: RRV000
HOSTS 2024-09-02
Most Rosa spp. and cultivated varieties such as climbers, hybrid teas, floribundas, and miniature roses are susceptible, at variable levels, to the disease (Claros et al., 2022). However, at the light of ongoing genetic studies seeking resistance to rose rosette disease, it appears that wild rose species native to North America and some from Asia may be a source of resistance to the rose rosette disease. In fact, although no complete resistance to rose rosette disease has been found among the major commercial rose cultivars, high levels of resistance have been found in a few cultivars with parentage originating from North American rose species (R. acicularis, R. arkansana, R. blanda, R. californica, R. carolina, R. palustris, R. pisocarpa, and R. setigera) and Asian rose species (R. spinosissima, R. wichuraiana, and R. bracteata). Most of these species and species hybrids have not been significantly explored for commercial breeding (Hochhaus et al., 2023; Amrine et al., 2002).
Mechanical inoculation tests resulted in the detection of RRV in newly developed leaves of tomato, pepper, Nicotiana spp., cucumber, squash, courgette (zucchini), pumpkin, pea, peanut, soybean, spinach, okra, and Chenopodium spp. by RT-PCR, but no severe symptoms were detected. Chenopodium spp., spinach, cucumber, and Nicotiana rustica developed mild chlorotic or necrotic lesions with variable shapes and patterns on systemically infected leaves (Atallah et al., 2022). The lack of notable, rapidly developed, characteristic symptoms hamper these hosts' use as diagnostic indicator plants.
Host list: Rosa arkansana var. suffulta, Rosa arkansana, Rosa banksiae, Rosa bracteata, Rosa canina, Rosa dumetorum, Rosa foliolosa, Rosa fortuniana, Rosa glauca, Rosa hybrids, Rosa multiflora, Rosa nutkana, Rosa pisocarpa, Rosa roxburghii, Rosa rubiginosa, Rosa rugosa, Rosa soulieana, Rosa spinosissima, Rosa villosa, Rosa wichuraiana, Rosa woodsii, Rosa x odorataGEOGRAPHICAL DISTRIBUTION 2024-09-02
RRV is reported to be epidemic in North America, in the USA and Canada (Bahari, 2015; Anon., 2024) and emerging in India (Chakraborty et al., 2017). RRV is not reported elsewhere.
North America: Canada (Manitoba, Ontario), United States of America (Alabama, Arizona, Arkansas, California, Connecticut, Delaware, District of Columbia, Florida, Georgia, Idaho, Illinois, Indiana, Iowa, Kansas, Kentucky, Louisiana, Maryland, Massachusetts, Michigan, Minnesota, Mississippi, Missouri, Nebraska, Nevada, New Hampshire, New Jersey, New Mexico, New York, North Carolina, Ohio, Oklahoma, Pennsylvania, Rhode Island, South Carolina, Tennessee, Texas, Utah, Virginia, Washington, West Virginia, Wisconsin, Wyoming)
BIOLOGY 2024-09-02
The biology and epidemiology of RRV are closely related to that of its eriophyid mite vectors: Phyllocoptes fructiphilus, the most studied species, and the recently described species, P. arcani sp. nov. (Druciarek et al., 2023). This section focuses on the overlapping biology of RRV and P. fructiphilus on Rosa spp. During the spring-summer growing season, viruliferous P. fructiphilus can reach the host by wind or other mechanical means. P. fructiphilus can be found sheltering under bud scales and on petals (T. Druciarek, pers. comm.), growing shoot tips (Hoy, 2013), within leaf folds of new shoots, or at petiole bases (Babu et al., 2015, citing others). The development from egg to adult takes approximately 11 days at 23°C (Kassar and Amrine, 1990). Multiple generations are then produced until the weather turns cold in the autumn; in mild weather, development may occur into the winter months (Tuffen, 2016, citing Amrine, 1996; Hoy, 2013). During the winter, females overwinter until early spring, hiding in protected inner plant places, such as beneath the bark or bud scales, on living host tissue (Babu et al., 2015, citing others). However, under greenhouse conditions P. fructiphilus generations are continuously produced without overwintering (T. Druciarek, pers. comm.). Passive aerial dispersal has been suggested as the primary way that eriophyids spread (Michalska et al., 2010; Sabelis and Bruin, 1996). This mode of spreading is also characteristic of P. fructiphilus. Although the maximum distance P. fructiphilus can spread by wind is unknown.
Transmission
RRV is transmitted by P. fructiphilus and P. arcani, as described above and by grafting (Di Bello, 2015; Di Bello et al., 2017). RRV vegetative propagation was suggested by Baker et al. (2014) but not demonstrated, the observation by Doudrick (1984) that cuttings from infected plants are less likely to root weakens the hypothesis of RRV being spread through vegetative propagation. Mechanical transmission studies using crude extracts (Doudrick, 1984; Epstein and Hill, 1999), showed that 5 out of 123 inoculated rose plants developed symptoms when a chilled (4oC) buffer containing antioxidants was used. Transmission of RRV to adjacent plants through root grafting was hypothesized (Allington et al., 1968) but not demonstrated. In addition, it is not clear whether root grafting happens in roses (Ong et al., 2014). No evidence of RRV seed and soil transmission was found (Di et al., 1990; Epstein and Hill, 1995; Windham et al., 2016). There is no evidence to suggest that RRV is transmitted through pollen, and no specific studies or references supporting pollen transmission have been found. For the other emaraviruses described to date, no transmission by pollen has been reported (Mielke-Ehret and Mühlbach, 2012). Attempts to transmit the disease by dodder (Cuscuta campestris, C. gronovii and C. pentagona) failed due to the dodder not producing haustoria on rose (Doudrick, 1984; Epstein and Hill, 1995; Epstein and Hill, 1999).
DETECTION AND IDENTIFICATION 2024-09-02
Symptoms
The symptoms of rose rosette disease are highly variable depending on the rose species, cultivar, and plant development stage. Symptoms may vary within the same cultivar, whether they are grown in the same location or in different locations (Epstein and Hill, 1995; Windham et al., 2014 a,b). Rose rosette disease symptoms include rapid elongation of lateral shoots, thickening of shoots, reddening of leaves and shoots, leaves showing critical nutritional deficiencies, masses of shoot proliferation (witches’ broom), excessive thorns, malformation, reduced flowering, and deformed buds and flowers (Amrine et al., 1988; Epstein and Hill, 1995, Dobhal et al., 2016). Infected plants become severely disfigured within one to three years after infection, and they usually die before the end of the first winter after symptoms become apparent. Otherwise, plant death occurs after two to four years. The incubation period reported in the literature varies from a few weeks to over one year (Epstein and Hill, 1999; Di Bello et al., 2017; Tipping and Sindermann, 2000).
Morphology
Transmission electron microscopy has revealed double membrane-bound particles of 80–120 nm in diameter in mechanically inoculated infected tissues of cucumber, pepper, and N. benthamiana (Atallah et al., 2022). This observation is in agreement with the morphology of Fimoviruses, which have quasi-spherical, enveloped virions with a diameter of 80–150 nm (Digiaro et al., 2024).
Detection and inspection methods
RRV detection relies mainly on molecular tests. Different molecular and serological diagnostic methods have been developed, including Reverse Transcription (RT) conventional Polymerase Chain Reaction (PCR) and real-time RT-PCR, RT-Loop-mediated isothermal amplification (RT-LAMP), recombinase-polymerase amplification (RPA), primers for broad detection of emaraviruses and the eriophyid mite vector Phyllocoptes fructiphilus, ELISA, and high-throughput sequencing (HTS) using bioinformatic pipelines (Vazquez-Iglesias et al., 2020; Claros et al., 2022). RRV-infected plants can remain symptomless for long periods, so these diagnostic tests are necessary in conjunction with visual assessment of symptoms to speed up early detection (Vazquez-Iglesias et al., 2020). A synthetic, multitarget, clonable, and non-infectious artificial positive control (APC) was designed de novo to be inserted in a circular plasmid vector that is amplified by most RRV-reported primers, probes, including primers for P. fructiphilus. The APC insert consists of a tandem of primers designed mainly to amplify control sequences for RRV, which also amplify a control product for broad detection of emaraviruses and Phyllocoptes fructiphilus using various nucleic acid amplification methods, including RT-PCR, RT-real-time PCR, RT-LAMP, and RPA (Ruschel et al., 2023). The APC-RRV system is robust, not-infectious, and capable of being integrated into 1.2 mm paper matrices. The APC is cloneable and subjected to stringent quality control, making it suitable for quarantine surveillance and routine diagnostics of RRV. This approach reduces the need to handle and transport infected tissue samples (Ochoa-Corona, personal communication).
PATHWAYS FOR MOVEMENT 2024-09-02
RRV and its mite vectors are considered to be potentially associated with all Rosa species and cultivars. The main pathway for RRV and its mite vectors is the international movement of infected Rosa plants for planting (e.g. bare-rooted plants, potted plants, cuttings, rootstocks, and possibly tissue cultures). Pollen and seed are not considered as a potential pathways, as there are no reports of RRV detection in these plant parts. Rose cut flowers could also transport RRV and its vectors, however the possibility of finding flowers with rose rosette disease symptoms in the market is low because they will be discarded due to the high-quality selection standards of cut roses (EPPO, 2018, Vazquez-Iglesias et al., 2020; Claros et al., 2022; Hochhaus et al., 2023).
PEST SIGNIFICANCE 2024-09-02
Economic impact
RRV and its vector, the eriophyid mite P. fructiphilus, have had high economic and social impacts in the USA. All species and cultivars of Rosa are considered at risk from the virus and vector, as no known tolerant or resistant species or varieties have been released at present. In the USA, RRV constitutes a severe threat to the rose industry, which includes commercial nursery production, breeding, private gardens, landscaping services, and retailers. The severity of the rose rosette disease impact and broad distribution seen in the continental USA has been described as ‘an epidemic’ (Bahari, 2015) and subsequently as ‘endemic’ (Pemberton et al., 2018), which led the United States Department of Agriculture (USDA) to fund research to devise solutions to control the disease and prevent more damage.
Control
Once RRV infects plants, no curative treatment is available, so it is recommended that infected plants are removed before reaching an advanced final stage due to their unsightly appearance (Pemberton et al., 2018). Removal and destruction must include root systems, as RRV infection is systemic (Di Bello et al., 2017). This practice should also help reduce the mite population.
When removing plants, precautions should be taken to avoid the spread of P. fructiphilus and RRV, e.g. bagging removed plant material during transportation, not leaving removed plant material on the site (Windham et al., 2016). Removal of symptomatic plants is however not considered fully effective as asymptomatic plants may also be present (EPPO, 2018).
Not all authors agreed on the efficacy of measures aimed at controlling the mite, especially the use of acaricides. Mites tend to shelter in crevices which are difficult for products to reach (Cloyd, 2013, Hand, 2014). In addition, treatments will not entirely prevent transmission of the virus to healthy roses as the vector has a short inoculation access period (1h) (EPPO, 2018).
The efficacy of foliar applications of acibenzolar-S-methyl (ASM), a plant systemic acquired resistance inducer, in reducing rose rosette disease severity was studied at 50-100-mg/L of ASM concentrations in glasshouse conditions on Rosa species cv. Radtkopink (‘Pink Double Knock Out’). ASM delayed the incidence of rose rosette disease compared to nontreated controls after three trials. Overall, plants treated with ASM at the 50-mg/L concentration had 36 to 43% reduced disease incidence compared with the water control. Real-time RT-PCR assessed the RRV presence. Treatment of two cultivars of rose, ‘Radtkopink’ and ‘Meijocos’ (‘Pink Drift’), with weekly foliar applications of ASM showed no adverse effect on flowering and plant growth (Babu et al., 2022).
In the USA and Canada, IPM strategies are being developed to contain the disease and usually include:
Genetic studies are under way to identify resistance genes in rose populations. Quantitative trait loci (QTL) for reduced susceptibility to rose rosette disease have been localized in tetraploid and diploid rose populations for marker-based selection to track and use a given QTL in plant breeding against rose rosette disease (Hochhaus et al., 2023).
Phytosanitary risk
Roses are widely planted in the EPPO region, especially in gardens, landscaped areas, tourist sites, and are also a valued nursery product. If RRV was introduced into the EPPO region, the highest economic impacts could be expected in nurseries and areas producing rose products, such as rose oil and pharmaceuticals. Potential environmental impacts are expected if native (especially endangered) Rosa species are infected in regions populated with susceptible hosts. Social impacts would occur through the loss of employment and income in the production and processing industry (especially for rose flowers for oil) and in those countries where Rosa has significant cultural importance.
The risk of entry on Rosa plants for planting (except seeds and pollen) is considered high with moderate uncertainty. The likelihood of establishment in regions where roses grow well is high because of the high volume of international trade and movement of people. If RRV and vector are introduced into the EPPO region the spread would be moderate to high due to the extensive trade in Rosa and the aerial dispersal of P. fructiphilus, with moderate uncertainty (EPPO, 2018).
PHYTOSANITARY MEASURES 2024-09-02
Considering the severe damage caused by RRV to roses, avoiding its introduction into the EPPO region is desirable. Effective phytosanitary measures should include monitoring and early detection of infections, destruction of RRV-infected plant material, and control of mite populations. Quarantine regulations and restrictions on the movement of rose plants from areas where rose rosette and mite vectors were reported are essential. It can be recommended that rose plants for planting and cut flowers should originate from pest-free areas for RRV, P. fructiphilus, and P. arcani and have been packed in conditions preventing mite infestation during transport (EPPO, 2018).
REFERENCES 2024-09-02
Allington W, Staples R & Viehmeyer G (1968) Transmission of rose rosette virus by the eriophyid mite Phyllocoptes fructiphilus. Journal of Economic Entomology 61, 1137-1140.
Amrine J (1996) Phyllocoptes fructiphilus and biological control of multiflora rose. In Lindquist EE, Sabelis MW & Bruin J (eds) World Crop Pests 6, Elsevier, pp. 741-749.
Amrine JW (2002) Multiflora rose. In Van Driesche R, Blossey B, Huddle M, Lyon S & Reardon R (eds) Biological Control of Invasive Plants in the Eastern United States. FHTET-2002-04; USDA, Forest Service: Washington, DC, USA; pp. 272–309.
Amrine JW, Hindal DF, Stasny TA, Williams RL & Coffman CC (1988) Transmission of the rose rosette disease agent to Rosa multiflora by Phyllocoptes fructiphilus (Acari, Eriophyidae). Entomological News 99, 239-252.
Anonymous (2024) Sustainable Roses. Developing sustainable roses through education and breeding. https://roserosette.org (consulted 2024-06-07).
Atallah OO, Yassin SM, Shirley N & Verchot J (2022) Exploring the host range of Rose rosette virus among herbaceous annual plants. Pathogens 11(12), 1514. https://doi.org/10.3390/pathogens11121514
Baker C, Schubert T, Srivastava P, Paret M & Babu B (2014) Rose rosette disease (Rose rosette virus) found in Florida. Florida Department of Agriculture and Consumer Services, Florida, USA. Available at: https://ccmedia.fdacs.gov/content/download/35585/file/rose%20rosette%20disease.pdf
Babu B, Paret ML, Martini X, Knox GW, Riddle B, Ritchie L, Aldrich J, Kalischuk M & Da Silva S (2022) Impact of foliar application of acibenzolar-S-methyl on rose rosette disease and rose plant quality. Plant Disease 106(3), 818-827. https://doi.org/10.1094/PDIS-01-21-0131-RE
Babu B, Paret ML, Schubert T, Baker C, Knox G, Iriarte F, Aldrich J, Ritchie L, Harmon CL & Folimonova SY (2015) Rose rosette disease: A new disease of roses in Florida: PP317/PP317, 5/2015. EDIS 2015 (4). Gainesville, FL, 6. https://doi.org/10.32473/edis-pp317-2015.
Bahari S (2015) Rose rosette is an epidemic, and North Texas is the epicenter. Fort Worth Star-Telegram, July 20,2015.
Chakraborty P, Das S, Saha B, Karmakar A, Saha D & Saha A (2017) Rose rosette virus: An emerging pathogen of garden roses in India. Australasian Plant Pathology 46, 223. https://doi.org/10.1007/s13313-017-0479-y
Claros NA, Shires M, Mollov D, Hammond J, Jordan R, Ochoa-Corona F, Olson J, Ong K & Salamanca LR (2022) Rose rosette disease: A diagnostic guide. Plant Health Progress 23(4), 482-491. https://doi.org/10.1094/PHP-05-22-0047-DG
Cloyd RA (2013) Rose rosette disease. MF2974. Kansas State University Agricultural Experiment Station and Cooperative Extension Service, 2 pp. Available at: https://bookstore.ksre.ksu.edu/pubs/rose-rosette-disease_MF2974.pdf (accessed 2024-06-07).
Di Bello PL, Ho T & Tzanetakis IE (2015) The evolution of emaraviruses is becoming more complex: seven segments identified in the causal agent of Rose rosette disease. Virus Research 210, 241-244.
Di Bello PL, Thekke‐Veetil T, Druciarek T & Tzanetakis IE (2017) Transmission attributes and resistance to rose rosette virus. Plant Pathology 67(2), 499-504.
Di R, Hill J & Epstein A (1990) Double-stranded RNA associated with the rose rosette disease of multiflora rose. Plant Disease 74, 56-58.
Digiaro M, Elbeaino T, Kubota K, Ochoa-Corona FM & von Bargen S (2024) ICTV virus taxonomy profile. Journal of General Virology 105, 00194. https://doi.org/10.1099/jgv.0.001943
Dobhal S, Olson JD, Arif M, Garcia Suarez JA & Ochoa-Corona FM (2016) A simplified strategy for sensitive detection of Rose rosette virus compatible with three RT-PCR chemistries. Journal of Virological Methods 232, 4756.
Dobhal S, Olson JD, Arif M, Garcia Suarez JA & Ochoa-Corona FM (2016) A simplified strategy for sensitive detection of Rose rosette virus compatible with three RT-PCR chemistries. Journal of Virological Methods 232, 47-56. https://doi.org/10.1016/j.jviromet.2016.01.013
Doudrick RL (1984) Etiological studies of rose rosette. MS thesis, University of Missouri-Columbia library, 101 pp.
Druciarek T, Lewandowski M &Tzanetakis I (2023) Identification of a second vector for Rose rosette virus. Plant Disease 107 (8), 2313-2315. https://doi.org/10.1094/PDIS-11-22-2686-SC
EPPO (2018) Pest risk analysis for Rose rosette virus and its vector Phyllocoptes fructiphilus. EPPO, Paris. Available at https://gd.eppo.int/taxon/RRV000/documents
Epstein A & Hill J (1995) The biology of rose rosette disease: A mite-associated disease of uncertain aetiology. Journal of Phytopathology 143, 353-360.
Epstein A & Hill J (1999) Status of rose rosette disease as a biological control for multiflora rose. Plant Disease 83, 92-101.
Hand F (2014) Controlling Rose Rosette Disease. In The Buckeye. Ohio Nursery and Landscape Association, Ohio, USA, pp. 37-40. Available at: https://chadwickarboretum.osu.edu/sites/chadwick/files/imce/pdf/Buckeye_08August2014_Hand.pdf (accessed 2024-06-07).
Hoy M (2013) Common name: eriophyid mite vector of rose rosette disease (RRD). Scientific name: Phyllocoptes fructiphilus Keifer (Arachnida: Acari: Eriophyidae). University of Florida, Florida, USA. Available at: http://entnemdept.ufl.edu/creatures/ORN/ph_fructiphilus.htm (accessed 2024-06-07).
Kassar A & Amrine JW (1990) Rearing and development of Phyllocoptes fructiphilus (Acari: Eriophyidae). Entomological News 101(5), 276-282.
Michalska K, Skoracka A, Navia D & Amrine JW (2010) Behavioural studies on eriophyoid mites: an overview. Experimental and Applied Acarology 51, 31-59. https://doi.org/10.1007/s10493-009-9319-2
Mielke-Ehret N & Mühlbach HP (2012) Emaravirus: a novel genus of multipartite, negative strand RNA plant viruses. Viruses 4, 1515-1536.
Ong K, Giesbrecht M & Woodson D (2014) Rose rosette demystified. Texas A&M, Agrilife Extension, 5 pp.
Pemberton HB, Ong K, Windham M, Olson J & Byrne DH (2018) What is rose rosette disease? HortScience 53(5), 592-595. https://doi.org/10.21273/HORTSCI12550-17
Ruschel RG, Taylor M, Ochoa-Corona FM, Amirudeen AKJ, Druciarek T & Paret M (2023) An artificial positive control for routine detection of rose rosette virus and Phyllocoptes fructiphilus that fit most primers for PCR, LAMP and RPA based assays. Annals of Applied Biology 183(1), 67–79. https://doi.org/10.1111/aab.12834
Sabelis MW & Bruin J (1996) Evolutionary ecology: life history patterns, food plant choice and dispersal. In: Lindquist EE, Sabelis MW & Bruin J (eds) Eriophyoid Mites – Their Biology, Natural Enemies and Control. Elsevier Science Publishing, Amsterdam, 329–366.
Tuffen M (2016) Rapid Pest Risk Analysis (PRA) for: Rose rosette virus and its vector Phyllocoptes fructiphilus. Department for Environment, Food and Rural Affairs (DEFRA), UK.
Vazquez-Iglesias I, Ochoa-Corona FM, Tang J, Robinson R, Clover GRG, Fox A & Boonham N (2020) Facing Rose rosette virus: A risk to European rose cultivation. Plant Pathology 69(9), 1603-1617. https://doi.org/10.1111/ppa.13255
Walker PJ, Siddell SG, Lefkowitz EJ et al. (2021) Changes to virus taxonomy and to the International Code of Virus Classification and Nomenclature ratified by the International Committee on Taxonomy of Viruses (2021). Archives of Virology 166, 2633–2648. https://doi.org/10.1007/s00705-021-05156-1
Windham M, Windham A & Hale F (2014a) Observations on Rose Rosette Disease. University of Tennessee (US). Available at: http://www.newenglandgrows.org/pdfs/ho_WindhamRoseRosette.pdf (accessed on 2024-06-07).
Windham M, Windham A, Hale F & Hitch W (2014b) Rose rosette: Identification and management. SNA Research Conference 59, 143–146. https://rose.org/wp-content/uploads/2024/05/W833-compressed.pdf
Windham M, Windham A & Hale F (2016) Observations on Rose Rosette Disease. The University of Tennessee. Available at: http://www.newenglandgrows.org/pdfs/ho_WindhamRoseRosette.pdf
ACKNOWLEDGEMENTS 2024-09-02
This datasheet was prepared in 2024 by Francisco M. Ochoa-Corona, Oklahoma State University, Institute for Biosecurity and Microbial Forensics. His valuable contribution is gratefully acknowledged.
How to cite this datasheet?
Datasheet history 2024-09-02
This datasheet was first published online in 2024. It is maintained in an electronic format in the EPPO Global Database. The sections on 'Identity', ‘Hosts’, and 'Geographical distribution' are automatically updated from the database. For other sections, the date of last revision is indicated on the right.
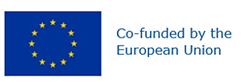