Rhynchophorus palmarum(RHYCPA)
EPPO Datasheet: Rhynchophorus palmarum
IDENTITY
Authority: (Linnaeus)
Taxonomic position: Animalia: Arthropoda: Hexapoda: Insecta: Coleoptera: Dryophthoridae
Other scientific names: Calandra palmarum (Linnaeus), Cordyle barbirostris Thunberg, Curculio palmarum Linnaeus, Rhynchophorus barbirostris (Thunberg), Rhynchophorus cycadis Erichson, Rhynchophorus depressus Chevrolet, Rhynchophorus languinosus Chevrolet
Common names in English: South American palm weevil, giant palm weevil, palm marrow weevil, palm marrow weevil, palm weevil
view more common names online...
EPPO Categorization: A1 list
EU Categorization: A1 Quarantine pest (Annex II A)
view more categorizations online...
EPPO Code: RHYCPA
HOSTS 2020-09-01
R. palmarum has been reported on at least 35 plant species in at least 12 different families. However, it is primarily found in association with palms (Arecaceae) (Esser & Meredith, 1987; Griffith, 1987; Wattanapongsiri, 1966; Jaffé & Sánchez, 1990; Sánchez & Cerda, 1993). With respect to palms, R. palmarum is commonly reported as a pest. It has also been observed reproducing on sugarcane stems, a non-palm host, (Arango & Rizo, 1977; Restrepo et al., 1982). Feeding damage by larvae to the apical meristematic region (i.e. the palm heart) causes palm mortality (Milosavljević et al., 2019). When reported on other plants, R. palmarum adults were observed feeding on ripe fruit. This type of feeding on fruit by adult weevils is typically not a source of significant economic damage.
Breeding hosts: R. palmarum breeding is primarily restricted to palms (Arecaceae) and known hosts include: Brahea edulis (Guadalupe palm), Cocos nucifera (coconut), Dypsis lutescens (golden cane palm), Elaeis guineensis (African oil palm), Euterpe edulis (juçara, grown for hearts of palm), Livistona australis (cabbage tree palm), Metroxylon sagu (true sago palm), Phoenix canariensis (Canary Islands date palm), P. dactylifera (edible date palm), and Washingtonia robusta (Mexican fan palm). A notable non-palm host used for reproduction is Saccharum officinarum (sugarcane [Poaceae]).
Adult food hosts: Adult R. palmarum feed on a variety of ripe fruit, including avocado (Persea americana [Lauraceae]), banana (Musa spp. [Musaceae]), Citrus spp. (Rutaceae), cocoa (Theobroma cacao [Malvaceae]), guava (Psidium guajava [Myrtaceae]), mango (Mangifera indica [Anacardiaceae]), and papaya (Carica papaya [Caricaceae]). These hosts are not used for reproduction instead the fruit provide nutrition and energy to adult weevils. Adult R. palmarum can be kept alive in laboratory colonies for several months on a diet of cut apple pieces and banana chunks that have the skin left on. Adult weevils will burrow into the banana pulp to hide and feed.
Artificial diets and laboratory rearing: Rearing of R. palmarum eggs and larvae for use in laboratory bioassays is possible with the use of artificial diets (Santana et al., 2014). A mixed diet of sugarcane and pineapple has been used to artificially rear R. palmarum (Giblin-Davis et al., 1991).
Host list: Acrocomia aculeata, Arecaceae, Attalea cohune, Attalea maripa, Bactris gasipaes, Bactris major, Brahea edulis, Chamaedorea elegans, Chrysalidocarpus decaryi, Chrysalidocarpus lutescens, Cocos nucifera, Elaeis guineensis, Euterpe edulis, Euterpe oleracea, Gynerium sagittatum, Howea forsteriana, Hyophorbe lagenicaulis, Jubaea chilensis, Livistona australis, Manicaria saccifera, Mauritia flexuosa, Metroxylon sagu, Oenocarpus bataua, Phoenix canariensis, Phoenix dactylifera, Phoenix reclinata, Phoenix roebelenii, Rhapis excelsa, Roystonea oleracea, Roystonea regia, Sabal bermudana, Sabal palmetto, Saccharum officinarum, Syagrus coronata, Syagrus orinocensis, Syagrus romanzoffiana, Syagrus schizophylla, Syagrus vagans, Washingtonia robustaGEOGRAPHICAL DISTRIBUTION 2020-09-01
The native range includes tropical parts of Mexico (e.g. Veracruz), Central (e.g. Costa Rica) and South America (e.g. Brazil and Colombia), and the Caribbean (e.g. Trinidad and Tobago). Despite the tropical distribution of this pest it has managed to invade distinctly non-tropical climates, most notably, southern California, USA. Invasion of arid semi-desert regions has been facilitated by human modification of the landscape. This modification includes irrigation of areas that are normally dry and the planting of a wide diversity and high density of palm species in urban areas where they do not naturally exist. In some instances, such as the desert-like Coachella Valley in California, commercial plantations of P. dactylifera may provide a suitable habitat for R. palmarum should it reach this area.
Invasion and establishment in California USA
Following the detection of R. palmarum infesting W. robusta in Todos los Santos in Baja California Sur (Mexico) in November 2000 (Garcia-Hernandez et al., 2003), this pest was found in December 2010, infesting P. canariensis in Tijuana, Baja California Norte, Mexico approximately 1,500 km north of Todos los Santos, and less than 3 km from the US border with San Diego County California. In the USA, adult R. palmarum were trapped in San Ysidro, California (approximately 5 km north of Tijuana) in May 2011; Alamo, Texas in March and May 2012; and Yuma, Arizona in May 2015. Established populations in San Ysidro were confirmed in 2015 (it is likely that populations had established earlier, perhaps in 2013-2014) and weevils have now established throughout large areas of San Diego County where they have killed thousands of ornamental P. canariensis in urban areas and naturalized P. canariensis in riparian areas. As of July 2020, there were no reports of established populations of R. palmarum in Arizona and Texas or palm mortality caused by this weevil. Locations of potential source populations for R. palmarum trapped in Arizona and Texas were not identified. Bursaphelenchus cocophilus, the red ring nematode, has not been detected in association with weevils captured in California, Texas, and Arizona.
Central America and Caribbean: Barbados, Belize, Costa Rica, Cuba, Dominica, Dominican Republic, El Salvador, Grenada, Guadeloupe, Guatemala, Haiti, Honduras, Martinique, Nicaragua, Panama, Puerto Rico, Saint Lucia, Saint Vincent and the Grenadines, Trinidad and Tobago
South America: Argentina, Bolivia, Brazil (Acre, Alagoas, Amazonas, Bahia, Ceara, Distrito Federal, Maranhao, Mato Grosso, Mato Grosso do Sul, Minas Gerais, Para, Paraiba, Parana, Pernambuco, Piaui, Rio de Janeiro, Rio Grande do Norte, Rio Grande do Sul, Roraima, Sao Paulo, Sergipe), Colombia, Ecuador, French Guiana, Guyana, Paraguay, Peru, Suriname, Uruguay, Venezuela
BIOLOGY 2020-09-01
Life cycle
In the native range, R. palmarum is commonly found in urban areas, forests, and oil palm plantations. In invaded regions in southern California, R. palmarum is largely restricted to urban areas where it attacks ornamental palms (e.g. P. canariensis, and to a lesser extent, B. edulis). In the native range, the altitudinal range is from sea level up to 1200 m (Jaffé & Sánchez, 1990). Studies on the biology of this species are reported in Wilson (1963), Nadarajan (1988), Sánchez et al. (1993) and Hagley (1965). The larvae of R. palmarum feed exclusively on live palm tissue in the meristematic region. Under laboratory conditions (20–35°C and 62–92% relative humidity), a female may lay an average of 245 ± 155 eggs during a period of 30.7 ± 14.3 days. The egg incubation period is 3.2 ± 0.93 days and larvae have between six and 10 instars which develop over a period of 52.0 ± 10.0 days. The prepupal stage lasts 4–17 days, during which larvae make cocoons using palm fibres. The pupal stage lasts 8–23 days and adults remain in cocoons for 7.8 ± 3.4 days before emerging. Adult males live for 44.7 ± 17.2 days and females for 40.7 ± 15.5 days. Hagley (1965) reported that a single female may lay up to 718 eggs, whereas Sánchez et al. (1993) reported a maximal oviposition of 697 eggs. Nadarajan (1988) and Sánchez et al. (1993) studied the biology of R. palmarum using artificial diets, and reported on mating and oviposition behavior observed in the laboratory.
Females can bore into palms with the rostrum to make oviposition holes. Females may also exploit damage wounds (e.g. pruning wounds or holes made by feeding insects) near or on the internodal area of the palm trunk next to the crown for oviposition. Eggs are oviposited into these holes. The egg rests in a vertical position in the hole which is sealed by the female with a secretion.
Adults are active during the day showing a bimodal daily activity cycle. Hagley (1965) reported major activity peaks between 07:00 and 11:00, and 17:00 and 19:00. Sánchez & Jaffé (1993) observed flight activity in the field, confirming the nature of the activity cycle, in which adults fly during the day, but avoid the hottest hours at noon and the early afternoon. Field observations showed that adults may fly at velocities of up to 6.01 m s−1 (Hagley, 1965). Studies on the population dynamics of this species in Central America are reported by Chinchilla (1988), showing that the maximum adult population occurs during the dry season. Similar results were obtained by Schuiling & Van Dinther (1981) in Brazil.
Vector of red ring nematode
The threat caused by R. palmarum is amplified by its ability to acquire and vector a plant pathogenic nematode, Bursaphelenchus cocophilus, the causative agent of red ring disease which is lethal to palms (Griffith 1987; Gerber and Giblin-Davis 1990; Oehlschlager 2016). Nematodes may be phoretic and attached to outside of the weevil or they can be introduced into palms by adults when feeding or defecating. No other species of Rhynchophorus vector plant pathogenic nematodes. Up to 90% of adult R. palmarum collected from palms with red ring disease are infected with B. cocophilus (Gerber and Giblin-Davis, 1990) and nematode infections in coconut and oil palms in the neotropics result in annual losses of 10-15% from red ring disease (Giblin-Davis et al., 2013). B. cocophilus is not vector-specific and can be spread by other species of weevils (e.g. Dynamis borassi) making it likely that other species of Rhynchophorus (e.g. the highly invasive red palm weevil, R. ferrugineus) could acquire and vector B. cocophilus should they become sympatric (Giblin-Davis et al., 2013).
DETECTION AND IDENTIFICATION 2020-09-01
Symptoms
Weevil larvae feed in the palm crown which can kill the apical growing region. This feeding causes the central area of the crown to tilt then collapse, as this is happening fronds from this central region begin to fall from infested palms. This results in a halo of dying mature fronds encircling the top of the palm trunk. Basal sheaths that are found at the bottom of fronds are often perforated with large holes when weevil infestations are heavy. With respect to P. canariensis, the basal areas of dropped fronds can be heavily tunneled, and often pupal cases can be found tightly wedged into tunnels. When infestations are especially heavy, pupal cases may drop from palms and can be readily found on the ground under infested palms. In parts of the native range, Dynamis borassi, a sympatric and morphologically similar looking weevil, may cause similar damage (Jaffé et al., 1993; Chinchilla & Öhlschlager, 1992, 1993; Sánchez & Jaffé, 1993).
Morphology
The morphology of R. palmarum eggs, larvae, pupae, and adults are described by Wattanapongsiri (1966). A summary is provided below.
Eggs
Eggs are 1–2 mm in length and are laid inside soft plant tissue, near the apical area of the palm. Females seal oviposition wounds with a waxy secretion presumably to protect eggs. Eggs are white and oblong in shape when recently laid. As eggs mature they may exhibit undulatory movements as larvae prepare to emerge. Around this time larvae can be seen through the egg chorion.
Larvae
The larvae are legless and creamy white, they may reach 5–6 cm in length prior to pupation. Larvae are cannibalistic and possess sclerotized mouth parts with strong mandibles. Their body is slightly curved ventrally. Prepupal larvae become darker and migrate from feeding sites to areas (e.g. base of the palm frond) where they will excavate a pupal chamber within which the fibrous pupal cocoon is spun.
Pupae
Pupae are exarate and light brown. The abdomen continuously makes undulatory movements when disturbed. Pupae inhabit a cylindrical-ovoid cocoon that is built with strongly entwined palms fibres. The fibrous cocoon is approximately 7 cm long and 3–4 cm in diameter.
Adults
Adult R. palmarum are typically black. However, a small proportion of the population may exhibit orange and black markings that are very similar in appearance to R. ferrugineus (Löhr et al., 2015). Adults are large, measuring 4–5 cm in length and are approximately 1.4 cm wide, weighing 1.6–2 g. The head is small with a characteristic long, ventrally curved rostrum. Adults show sexual dimorphism; males have a conspicuous batch of hairs on the antero-central dorsal region of the rostrum. Females lack rostral setae.
The morphological identification of R. palmarum is described in the EPPO diagnostic protocol for Rhynchophorus ferrugineus and Rhynchophorus palmarum (EPPO, 2007). Use of computer based tools (e.g. online keys, digital cameras attached to microscopes) and internet connectivity to access data bases, images, and experts via screen and image sharing software can aid in the rapid identification of Rhynchophorus spp. (Giblin-Davis and Roda, 2013).
Detection and inspection methods
Early detection of weevil infestations within palms is extremely difficult and often infestations are only confirmed once damage becomes visually obvious. Some early detection methods have explored the use of molecular and biochemical markers that palms or weevils produce as a result of attack. Another approach has been to use sensitive electronic equipment to ‘listen’ for acoustic signatures that typify weevil feeding. Changes in infrared spectral qualities of palms have also been assessed for diagnostic potential. Overall, early detection techniques have not been developed to a point where they are sufficiently accurate, cost effective, or easy and fast to use enabling the examination of many tens or hundreds of palms a day. Visual inspections for weevil infestations of small palms in containers is possible, but this approach is difficult for tall palms. Visual inspections of tall mature palms with binoculars can be done and drones flying over and around palms can provide high resolution images of the crown region for examination. However, accurately assigning the cause of frond damage (e.g. clipped ends of expanding fronds or notches) can be difficult as feeding damage by rats, for example, to immature fronds prior to expansion, can produce damage similar to that caused by R. palmarum.
PATHWAYS FOR MOVEMENT 2020-09-01
The pest can be accidentally spread over long distances through the movement of infested palms for planting into new areas. Adult weevils are capable of localized short distance flights and long distance flight may be possible. Flight mill studies in the laboratory suggest that R. palmarum is a strong flyer. The average distance flown by males and females over a 24 hr period is approximately 41 km and 53 km, respectively (Hoddle et al., 2020). In these flight mill studies, approximately 10% of females flew > 100 km in 24 h, with two (~4%) females flying > 140 km. The maximum recorded distance flown by a male weevil was 95 km. Flight activity was predominantly diurnal and flying weevils exhibited an average weight loss of approximately 18% over a 24 hr period (Hoddle et al., 2020). It is unknown if R. palmarum undertakes long distance flights in nature. However, flight mill data suggest strongly that they are capable of flying long distances. This possibility should be carefully considered when establishing quarantine boundaries or setting up detection and monitoring programs.
PEST SIGNIFICANCE 2020-09-01
Economic impact
R. palmarum is recognized as a serious pest of oil palms, coconuts, and ornamental palms. Countries reporting significant damage in oil palm (Elaeis guineensis) plantations include Central America (Costa Rica), Colombia, Venezuela and Brazil. (Griffith, 1968, 1970; Dean, 1979; Fenwick, 1967; Sánchez & Cerda, 1993). In Veracruz (Mexico), R. palmarum is a pest of ornamental palms (Landero-Torres et al., 2015). Economic tolerance for damage depends on the palm species and on the number of larvae infesting the plant. Fenwick (1967) and Griffith (1968) reported that as few as 30 R. palmarum larvae are sufficient to cause the death of a mature coconut palm.
In addition to direct damage, R. palmarum causes indirect damage by vectoring red ring nematode, B. cocophilus. R. palmarum was first suspected to vector B. cocophilus, a nematode responsible for heavy losses in coconut plantations, in the early 1900s (Cobb, 1922). It is now well established that R. palmarum is the principal vector of B. cocophilus, and that reduction in weevil population densities is key to lower nematode infestation. Coconut palms 3–10 years of age die during the first 2 months after inoculation with B. cocophilus (Griffith, 1987). Thurston (1984) and Brathwaite & Siddiqi (1975) reported that infected plants take 23–28 days to show the symptoms of red ring disease, and typically die 3–4 months after showing the first symptoms. Esser & Meredith (1987) estimated that several million USD are lost annually due to the association of red ring disease and R. palmarum. They estimated that 800 ha of coconut plantations were abandoned in 1923 due to this disease, and that in Grenada 22% of the coconut palms had red ring disease.
Although R. palmarum is undoubtedly a plant pest, in South America, it can provide some benefits to human communities by being used as a source of food or for traditional medicine. In parts of Amazonia, R. palmarum larvae are cultivated and marketed by indigenous groups (Choo et al., 2009; Delgado et al., 2019; Sancho et al., 2015).
Control
Trapping programs
Trapping programs utilize traps (e.g. bucket traps or cone shaped Picusan traps) loaded with commercially-available aggregation pheromone and baited with fermenting bait (e.g. sugarcane, dates and water, or pineapple chunks in syrup). The male produced aggregation has been identified as 2(E)-6-methyl-2-hepten-4-ol, (commonly known as rhynchophorol) (Rochat et al., 1991a,b; Öhlschlager et al., 1992a,b). Addition of ethyl acetate to traps loaded with pheromone and bait synergizes attractiveness (Jaffé et al., 1993). Recent studies by Milosavljević et al. (2020) demonstrated that ground deployed Picusan traps are 5 times more effective at catching R. palmarum than bucket traps that are suspended 1.5 m above the ground or placed on the ground. The design of the Picusan trap is significantly more effective at retaining weevils that enter this trap. The attractiveness of fermenting bait can be enhanced by the addition of yeast. Field trials demonstrated that baker’s yeast, Saccharomyces cerevisiae, added to dates and water produced volatiles that were more attractive than those produced by S. bayanus and S. pastorianus. However, the additional cost of yeast to boost bait fermentation may not be warranted. Weevil captures were statistically similar between traps baited with: (1) dates and water, and (2) dates, water, and S. cerevisiae. Traps with dates, water and S. bayanus or S. pastorianus caught significantly fewer weevils. Weevils attracted to traps can be killed by drowning through the addition of 50% propylene glycol solution. This is needed for bucket traps with holes that don’t prevent egress, but is probably unnecessary for Picusan traps as the funnel shaped ingress port effectively prevents egress after entry.
Mass trapping of R. palmarum at 1 trap/5-ha significantly lowered the incidence of red ring nematode infection vectored by the weevil in commercial oil palm plantations in the Americas (Öhlschlager et al., 1993; Oehlschlager, 2016). With respect to ornamental palms in urban areas, trap placement with respect to palms of interest is a highly important consideration. If traps are placed too close to palms, weevils attracted to traps but not retained and killed in traps may attack nearby palms. If the goal of trapping is to detect R. palmarum activity in the general vicinity of palms of concern, then traps could be deployed outside the immediate area of interest, perhaps approximately 1 km away. If weevils are captured at this distance from the palms of concern, it likely indicates there is probably a risk of weevil attack and steps should be promptly considered and implemented to protect those palms.
Trap colour (i.e. white vs. black vs. yellow) does not significantly affect R. palmarum capture rates (Oehlschlager et al., 1993). In order to maximize trap efficacy, traps should be placed in areas with partial or full shade. Full sun exposure, especially during the hottest parts of the day, rapidly diminishes trap efficacy (Navarro-Llopis et al., 2018).
Biological control
Relatively little is known about the natural enemies associated with R. palmarum in its native range. However, one promising candidate is a parasitic fly, Billaea rhynchophorae (Diptera: Tachinidae), from Brazil that is reported to attack R. palmarum larvae and pupae (Moura et al., 1993; 2006). Very little is known about the biology and ecology of this fly but its population-level impacts on R. palmarum in Brazilian oil palm plantations appear to be significant with year round parasitism averaging 50% (range: 32-74%) and each parasitized R. palmarum pupa produces around 18 flies. Löhr (2013) provides a compelling argument for increased research into potential biocontrol agents attacking R. palmarum in the native range. Billaea spp. are obvious candidates in need of detailed study.
Sanitation
Removal and destruction of palms infested with R. palmarum can be done by mechanically chipping or grinding infested fronds and removal and destruction of infested crowns either by burying 1.5 m underground or cutting with a chainsaw and then mechanically chipping or grinding into small pieces. Chipping or grinding will kill larvae, pupae, and adults, and this process helps desiccate eggs. Burning infested palm crowns is difficult and not recommended. Palm trunks with crowns removed can be left standing. It is unlikely that these will be used for breeding by R. palmarum as the meristematic region, the preferred feeding site for larvae, is not available. Applications of contact insecticides to the tops of cut trunks that have had the crowns removed can be made. Insecticide residues will kill adult R. palmarum attracted by wound volatiles.
Insecticides
Insecticidal control of R. palmarum is difficult (Hagley, 1963). However, systemic insecticides applied as either trunk sprays or injections, soil injections or drenches, or as crown drenches are effective. If applied during the early stages of infestation, insecticides may be capable of ‘curing’ infested palms. A combination of systemic insecticides with different rates of movement in palms can be effective. A combination approach may use fast moving compounds that reach internal larval infestation sites first and slower moving compounds follow up to provide more persistent control. Systemic insecticides that have both insecticidal and nematicidal properties need evaluation as these types of products have the potential to provide protection against R. palmarum and B. cocophilus. Applications of contact insecticides to crown regions can provide some protection from adult weevils and can be used in combination with systemic insecticides. Cutting an observation ‘window’ into the crown may help with observations to determine treatment efficacy. ‘Windows’ are made by removing fronds at the base of the crown so access to the crown is possible. In extreme situations, all of the fronds can be removed from the crown region so that the top of the palm has a bulbous-looking appearance. Insecticide treatments can be applied to this ‘bulb’ and in the absence of fronds less insecticide is needed as there is reduced run off and applications are highly focused on the infested region.
IPM of R. palmarum
IPM manuals outlining control programs for R. palmarum in Brazil (Moura, 2017) and Colombia (https://www.ica.gov.co/getattachment/19e016c0-0d14-4412-af12-03eecfe398f2/Manejo-del-picudo--Rhynchophorus-palmarum-L--(Cole.aspx) are available.
Phytosanitary risk
R. palmarum presumably presents a significant risk to edible date palms in North Africa and the Middle East. This potential risk will be better understood should this weevil invade and establish in edible date growing regions of California (e.g. the Coachella Valley) and Arizona (e.g. the Bard Valley). R. palmarum is a serious problem afflicting ornamental palms, especially P. canariensis, in California. It is highly likely to threaten P. canariensis throughout the Mediterranean region and in the Canary Islands, the native range of this palm. The current problem with R. ferrugineus in these areas would be greatly amplified if R. palmarum invaded and carried with it B. cocophilus as it is likely that R. ferrugineus would be able to vector this palm killing nematode. R. palmarum could potentially be introduced into countries of the EPPO region through imported palms from infested areas.
PHYTOSANITARY MEASURES 2020-09-01
R. palmarum was added in 2005 to the EPPO A1 list, and endangered EPPO member countries are thus recommended to regulate it as a quarantine pest. Imported plants for planting of palms should originate in a pest-free area or come from a pest-free place of production.
REFERENCES 2021-08-06
Arango G & Rizo D (1977) [Some considerations on the behaviour of Rhynchophorus palmarum and Metamasius hemipterus on sugarcane.] Revista Colombiana de Entomologia 3, 23-28 (in Spanish).
Brathwaite C & Siddiqi M (1975) Description of Rhadinaphelenchus cocophilus. In: Description of Plant-Parasitic Nematodes, no. 72. CAB International, Wallingford (GB).
Chinchilla C (1988) [The red ring/small leaf syndrome in oil palm and coconut.] Boletín Técnico Oil Palm Operations (Costa Rica) 2, 4 (in Spanish).
Chinchilla C & Öhlschlager AC (1992) Captures of Rhynchophorus palmarum in traps baited with the male-produced aggregation pheromone. ASD Oil Palm Papers 5, 1-8.
Chinchilla C & Öhlschlager AC (1993) [Traps for capture of adult Rhynchophorus palmarum, using the aggregation pheromone produced by the male.] Manejo Integrado de Plagas (Costa Rica) no. 29, 28-35 (in Spanish).
Choo J, Zent EL & Simpson BB (2009) The importance of traditional ecological knowledge for palm-weevil cultivation in the Venezuelan Amazon. Journal of Ethnobiology 29(1), 113-128.
Cobb NA (1922) A note on the coconut nematode of Panama. Journal of Parasitology 9, 44-45.
Dean C (1979) Red ring disease of Cocos nucifera caused by Rhadinaphelenchus cocophilus. An Annotated Bibliography and Review. CAB International, Wallingford (GB).
Delgado C, Romero R, Vásquez Espinoza R, Trigozo M, Correa R (2019) Rhynchophorus palmarum used in traditional medicine in the Peruvian Amazon. Ethnobiology Letters 10(1), 120–128.
EPPO (2007) EPPO standard PM 7/83 Diagnostics. Rhynchophorus ferrugineus and Rhynchophorus palmarum. EPPO Bulletin 37, 571-579.
Esser R & Meredith J (1987) Red ring nematode. Nematology Circular of the Florida Department of Agriculture no. 141, Gainesville (US).
Fenwick D (1967) The effect of weevil control on the incidence of red ring disease. Journal of the Agricultural Society of Trinidad and Tobago 67, 231-244.
Garcia-Hernandez JL, Beltran-Morales F, Loya-Ramirez JG, Morales-Cota JR, Troyo-Diéguez E, Beltrán-Morales EF (2003) Primer informe del Rhynchophorus palmarum (Coleoptera: Dryophthoridae) en Baja California Sur. Folia Entomológica Mexicana 42, 415-417.
Gerber K & Giblin-Davis RM (1990) Association of the red ring nematode and other nematode species with the palm weevil, Rhynchophorus palmarum. Journal of Nematology 22, 143-149.
Giblin-Davis RM, Gerber K & Griffith R (1991) Laboratory rearing of Rhynchophorus cruentatus and R. palmarum (Coleoptera: Curculionidae). Florida Entomologist 72(3), 480-488.
Giblin-Davis RM & Roda AL (2013) Real time invasive pest identification training: a case study with Rhynchophorus weevils. Florida Entomologist 96(3), 741-745.
Giblin-Davis RM., Faleiro JR, Jacas JA, Peña JE & Vidyasagar PSPV (2013) Biology and management of the red palm weevil, Rhynchophorus ferrugineus. In: Potential Invasive Pests of Agricultural Crops, Ed. J. E. Peña, pp. 1-34, CABI Publishing Oxfordshire, U.K.
Griffith R (1968) The relationship between the red ring nematode and the palm weevil. Journal of the Agricultural Society of Trinidad and Tobago 68, 342-356.
Griffith R (1970) Control of red ring disease in coconut. Crop Bulletin of the Ministry of Agriculture of Trinidad and Tobago 17, 1-3.
Griffith R (1987) Red ring disease of coconut palm. Plant Disease 71, 193-196.
Hagley E (1963) The role of the palm weevil as a vector of red ring disease of coconuts. Journal of Economic Entomology 56, 375-380.
Hagley E (1965) On the life history and habits of Rhynchophorus palmarum. Annals of the Entomological Society of America 58, 22-28.
Hoddle MS, Hoddle CD & Milosavljević I (2020) How far can Rhynchophorus palmarum (Coleoptera: Curculionidae) fly? Journal of Economic Entomology 113(4), 1786-1795. https://doi.org/10.1093/jee/toaa115
Jaffé K & Sánchez P (1990) [Final Report of Project on Ethological Study of Rhynchophorus palmarum.] Universidad Simón Bolívar-FONAIAP, Caracas (VE).
Jaffé K, Sánchez P, Cerda H, Hernández JV, Jaffé R, Urdaneta N, Guerra G, Martínez R & Miras B (1993) Chemical ecology of Rhynchophorus palmarum: attraction to host plants and to a male-produced aggregation pheromone. Journal of Chemical Ecology 19,
Landero-Torres I, Presa-Parra E, Elena Galindo-Tovar M, Leyva-Ovalle OR, Murguía-González J, Valenzuela-González JE & García-Martínez MA (2015) Variación temporal y espacial de la abundancia del picudo negro (Rhynchophorus palmarum L., Coleoptera: Curculionidae) en cultivos de palmas ornamentales del centro de Veracruz, México. Southwestern Entomologist 40(1), 179-188.
Löhr B (2013) Biological control of palm weevils: rediscovering forgotten opportunities. Biocontrol News and Information 34(3), 20N-23N.
Löhr B, Vásquez-Ordóñez AA & Becerra Lopez-Lavalle LA (2015) Rhynchophorus palmarum in disguise: Undescribed polymorphisms in the ‘black’ palm weevil. PLoS One 10(12), e0143210. https://do.org/10.1371/journal.pone.0143210
Milosavljević, I, El-Shafie HAF, Faleiro JR, Hoddle CD & Hoddle MS (2019) Palmageddon: the wasting of ornamental palms by invasive palm weevils, Rhynchophorus spp. Journal of Pest Science 92, 143-156.
Milosavljević I, Hoddle CD, Mafra-Neto A, Gomez-Marco F & Hoddle MS (2020) Effects of evaluation of food bait and trap type on captures of efficacy to lure and catch Rhynchophorus palmarum (Coleoptera: Curculionidae) and trap bycatch in Southern California. Journal of Economic Entomology https://doi.org/10.1093/jee/toaa175
Moura JIL, Mariau D & Delabie JHC (1993) Efficacy of Paratheresia menezesi for natural biological control of Rhynchophorus palmarum. Oléagineux 48, 219-223.
Moura JIL, Toma R, Sgrillo RB & Delabie JHC (2006) Natural efficiency of parasitism by Billaea rhynchophorae (Blanchard) (Diptera: Tachinidae) for the control of Rhynchophorus palmarum (L.) (Coleoptera: Curculionidae). Neotropical Entomology 35(2), 273-274.
Moura J (ed) (2017) Manejo Integrado das Pragas das Palmeiras. Ministério da Agricultura, Pecuária e Abastecimento Comissão Executiva do Plano da Lavoura Cacaueira. 186 pp.
Nadarajan L (1988) Laboratory rearing of the palm weevil Rhynchophorus palmarum on artificial diet. In: Rapport du Laboratoire des Médiateurs Chimiques. INRA, Versailles (FR).
Navarro-Llopis V, Primo J & Vacas S (2018) Improvements in Rhynchophorus ferrugineus (Coleoptera: Dryophthoridae) trapping systems. Journal of Economic Entomology 111,
Oehlschlager AC, Chinchilla CM, Gonzalez LM, Jiron LF, Mexzon R & Morgan B (1993) Development of a pheromone-based trapping system for Rhynchophorus palmarum (Coleoptera: Curculionidae). Journal of Economic Entomology 86,
Oehlschlager AC (2016) Palm weevil pheromones – discovery and use. Journal of Chemical Ecology 42, 617-630.
Öhlschlager A, Chinchilla C & González M (1992a) Management of Rhynchophorus palmarum and the red ring disease in oil palm by pheromone-based trapping. ASD Oil Palm Papers 5, 15-23.
Öhlschlager A, Pierce H, Morgan B, Wimalaratne P, Slessor K, King G, Gries G, Gries R, Borden J, Jirón L, Chinchilla C & Mexón R (1992b) Chirality and field testing of rhynchophorol, the aggregation pheromone of Rhynchophorus palmarum. Naturwissenschaften 79, 134-135.
Öhlschlager A, Chinchilla C, González L, Jirón L, Mexón R & Morgan B (1993) Development of pheromone-based trapping system for Rhynchophorus palmarum. Journal of Economic Entomology 86,
Restrepo L, Rivera F & Raigosa J (1982) Life cycle, habit and morphometry of Metamasius hemipterus and Rhynchophorus palmarum on sugarcane. Acta Agronómica 32, 33-44 (in Spanish).
Rochat D, Gonzalez A, Marian D, Villanueva A & Sagatti P (1991a) Evidence for male-produced aggregation pheromone in Rhynchophorus palmarum. Journal of Chemical Ecology 17,
Rochat D, Malosse C, Lettere M, Ducrot PH, Zagatti P, Renou M & Descoins C (1991b) Male-produced aggregation pheromone of Rhynchophorus palmarum: collection, identification, electrophysiological activity, and laboratory bioassay. Journal of Chemical Ecology 17,
Sánchez P & Cerda H (1993) [The complex Rhynchophorus palmarum / Bursaphelenchus cocophilus in palms.] Boletín de Entomología Venezolana 8, 1-18 (in Spanish).
Sánchez P, Jaffe K, Hernandez JV & Cerda H (1993) [Biology and behaviour of Rhynchophorus palmarum.] Boletín de Entomología Venezolana 8, 83-93 (in Spanish).
Sánchez P & Jaffé K (1993) [Monitoring and integrated control of Rhynchophorus palmarum on oil palm.] Boletín Técnico Serie B. FONAIAP, Caracas (VE).
Sancho D, Alvarez Gil MJ & Fernández Sánchez LR (2015) Insectos y alimentación. Larvas de Rhynchophorus palmarum L, un alimento de los pobladores de la Amazonía Ecuatoriana. Entomotropica 30(14), 135-149.
Santana CC, Nascimento JS, de M. Costa M, da Silva AT, Dornelas CB & Grillo LAM (2014) Avaliação do desenvolvimento e reservas energéticas de larvas de Rhynchophorus palmarum (Coleoptera: Curculionidae) em diferentes dietas. Agrária - Revista Brasileira de Ciências Agrárias 9(2), 205-209. https://doi.org/10.5039/agraria.v9i2a3885
Schuiling M & van Dinther J (1981) Red ring disease in the paricatuba oil palm estate, Brazil. Zeitschrift für Angewandte Entomologie 91, 154-169.
Thurston HD (1984) Red ring disease of coconut. In: Tropical Plant Diseases, pp. 161-164. American Phytopathological Society, St Paul (US).
Wattanapongsiri A (1966) A Revision of the Genera Rhynchophorus and Dynamis. Department of Agricultural Science, Bangkok (TH).
Wilson M (1963) Investigations into the development of the palm weevil, Rhynchophorus palmarum. Tropical Agriculture Trinidad 40, 185-196.
ACKNOWLEDGEMENTS 2020-09-01
This datasheet was extensively revised in 2020 by Mark Hoddle, Department of Entomology, University of California, Riverside California, USA. His valuable contribution is gratefully acknowledged.
How to cite this datasheet?
Datasheet history 2020-09-01
This datasheet was first published in the EPPO Bulletin in 2005 and is now maintained in an electronic format in the EPPO Global Database. The sections on 'Identity', ‘Hosts’, and 'Geographical distribution' are automatically updated from the database. For other sections, the date of last revision is indicated on the right.
EPPO (2005) Rhynchophorus palmarum. Datasheets on pests recommended for regulation. EPPO Bulletin 35(3), 468-471. https://doi.org/10.1111/j.1365-2338.2005.00883.x
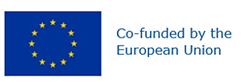