Diabrotica virgifera virgifera(DIABVI)
EPPO Datasheet: Diabrotica virgifera virgifera
IDENTITY
Authority: Leconte
Taxonomic position: Animalia: Arthropoda: Hexapoda: Insecta: Coleoptera: Chrysomelidae
Common names in English: Colorado corn rootworm, western corn rootworm
view more common names online...
Notes on taxonomy and nomenclature
Diabrotica virgifera was first described by LeConte in 1867 from blossoms of Cucurbita foetidissima in Western Kansas. Horn (1893) described Diabrotica filicornis from New Mexico, which was later recognized as males of D. virgifera (Mazzoni et al., 2007). Until 1980, no subspecies of Diabrotica virgifera were described. But following various observations on the distribution, morphology and biology of ‘Mexican populations’ of Diabrotica which are adapted to a tropical climate, Krysan et al. (1980) distinguished the subspecies Diabrotica virgifera zeae from the nominate subspecies Diabrotica virgifera virgifera LeConte (see also EPPO datasheet on D. virgifera zeae).
EU Categorization: Emergency measures (formerly)
view more categorizations online...
EPPO Code: DIABVI
HOSTS 2023-04-18
D. virgifera virgifera was thought to be dependent upon maize (Zea mays) for the completion of its immature stages (Branson and Ortman, 1970), with the larvae feeding on the roots. However, larvae feeding on other Poaceae are well documented by different authors. Laboratory tests have shown that the larvae can develop for example on Hordeum vulgare, Triticum aestivum, Triticum spelta or Oryzae sativa (Branson and Ortman, 1967; 1970). Wilson and Hibbard (2004) showed that larvae can survive on 20 non-maize grass plant species (and recovered adults on 10 of these species). Most of these species offer poor conditions for larval development resulting in high larval mortality and thus the percentage of adult emergence remains low, adults being of smaller average size (Ellsbury et al., 2005). But the proportion of D. virgifera virgifera immature stages surviving on non-maize hosts and their potential negative impact on maize fields in the immediate vicinity would need to be assessed in field conditions. Fora and Lauer (2013) have investigated this aspect in Romanian fields. They concluded that Digitaria sanguinalis, Echinochloa crus-galli, Elymus repens (synonym Agropyron repens), Cenchrus americanum (synonym Setaria glauca), Setaria verticillata and Setaria viridis are host plants for the pests but not Sorghum halepense, Triticum aestivum and Hordeum vulgare in the fields.
Adults feed on leaves (its preferred food according to Ludwig and Hill, 1975), silks and tassels of maize which are their primary food source. But after the depletion of those, they start to feed on weed pollen. In Hungary, pollen of 19 species of plants from nine families was found in the gut contents of D. virgifera virgifera (for example Asteraceae, Cucurbitaceae, Malvaceae, Poaceae) (Moeser, 2003). This pollen originated from flowering weeds within maize fields and a few from other crops such as sunflower and alfalfa. However, neither larvae nor adults have yet been reported to cause economic damage on crops other than maize in the EPPO region.
Host list: Cenchrus americanus, Digitaria sanguinalis, Echinochloa crus-galli, Elymus repens, Setaria verticillata, Setaria viridis, Zea maysGEOGRAPHICAL DISTRIBUTION 2023-04-18
D. virgifera virgifera was first described from the far west of Kansas, but is probably of tropical origin, and the species has adapted to continental climates (Lombaert et al., 2018). A review of historical records by Branson and Krysan (1981) led the authors to speculate that the insects moved during the prehistoric period, in the same period as maize spread. But current genetic studies indicate that the expansion of the species north of its origin was more recent—probably not before 1100 years ago— and as a consequence, not directly associated with the early history of maize expansion (Lombaert et al., 2018). Before it became a serious pest of maize in the 1940s, populations were low (Branson and Krysan, 1981) with only a report of damages on sweetcorn in North Central Colorado in 1909 (Gillette, 1912). Around 1940, D. virgifera virgifera was routinely damaging maize in South-Western Nebraska, and it became a problem in North-Western Kansas in 1945. From that date, the increased adoption of continuously planting maize without crop rotation created conditions suitable for rapid population growth, and thus increased the number of individuals dispersing from infested areas. D. virgifera virgifera then expanded its range across the Midwest, reaching West-Central Wisconsin by 1964, North-West Indiana by 1968 and the Atlantic Coast by the mid-1980s. D. virgifera virgifera is now established from Montana and North Dakota in the northwest, to Quebec and New England in the northeast, Northern Georgia and Alabama in the southeast (Guillemaud, 2007) and Mexico in the south. In Texas, D. virgifera virgifera and D. virgifera zeae are present together and beetles of intermediate appearance have already been collected in nature (Krysan et al., 1980).
In Europe, D. virgifera virgifera was first reported in 1992 in Serbia but mathematical simulations of the population growth from the year of introduction until 1992 indicate that the first successful introduction into Europe is likely to have occurred between 1979 and 1984 (Szalai et al., 2010) with the population source probably being Pennsylvania (Ciosi et al., 2008). D. virgifera virgifera has now reached various Western and South-Eastern European countries, from the United Kingdom to Bulgaria, and from Poland to Serbia. The current status of the pest varies in each country (Bazok et al., 2021). In some, the pest is widespread, in others, it is distributed across a limited area that corresponds to the area suitable for maize cultivation or is still limited to restricted regions.
North America: Canada (Ontario, Québec), Mexico, United States of America (Alabama, Arizona, Colorado, Connecticut, Delaware, Georgia, Idaho, Illinois, Indiana, Iowa, Kansas, Kentucky, Louisiana, Maine, Maryland, Massachusetts, Michigan, Minnesota, Missouri, Montana, Nebraska, New Hampshire, New Jersey, New Mexico, New York, North Carolina, North Dakota, Ohio, Oklahoma, Pennsylvania, Rhode Island, South Dakota, Tennessee, Texas, Utah, Virginia, Washington, West Virginia, Wisconsin, Wyoming)
BIOLOGY 2023-04-18
The bionomics of D. virgifera virgifera has been extensively studied by many authors, including Chiang (1973), Branson (1976), Krysan et al. (1977), Krysan (1978), Schaafsma et al. (1991) and Levine et al. (1992). D. virgifera virgifera is univoltine, overwintering as an egg in the soil. Eggs are generally oviposited in the top 15 cm of soil, at the base of maize plants, from July to September and development halts within about 11-13 days at 20°C as the egg enters diapause. Mean duration of diapause in natural populations (8–10 months) varies between studies and latitudes and is quite variable among individuals within a population. During this period, eggs are exposed to harsh conditions, resulting in overall high mortality. Termination of diapause does not require chilling or other known environmental signals, but is an event apparently governed by time. Diapauses termination occurs during midwinter when soil temperatures are still below 11°C, the thermal threshold for development. In addition to warm enough temperatures, post diapause eggs require uptake of water to complete development. Prolonged diapauses (eggs that terminate diapause only during the second winter) is known from laboratory experiments but only involves a very small proportion of the population and is not known to be responsible for the widespread root injury to rotated maize in eastern portions of the United States Corn Belt (Gray et al., 2009).
Eggs hatch at the beginning of the cropping season and the larvae develop in and on the roots of maize (and some other Poaceae, see the Host section), the young larvae feeding on fine rootlets and the older ones invading the root core. Larval development progresses through 3 instars and rate of development is temperature dependent. First instar larval movement and later establishment on maize roots is a critical stage in the life of the larvae, and the highest mortality is observed during this stage in the field (94% marginal death rate) (Toepfer and Kuhlmann, 2006). Neonate larvae use carbon dioxide for host location but larval movement and thus the success of their establishment depends also on texture, structure, and bulk density of the soil. In the United States Corn Belt, D. virgifera virgifera adult emergence begins in late June to early July with peak emergence often occurring during July. This general pattern is consistent with observations in Central and Eastern European countries. The rate of beetle emergence is positively correlated with spring temperatures according to the model of Jaksons et al. (2022). First emergence of males precedes that of females by ca. 5 days. Average female neonate larva to adult emergence times ranged from 45.0 - 20.7 days at 18 – 30°C. The lower thermal threshold for immature D. virgifera virgifera development is 9°C (Jackson and Elliott, 1988).
Adults mate, disperse and lay eggs during the summer. As maize grows, they feed on all above ground tissue of maize starting on the leaves, then on pollen and silk and then finally on the kernel, before moving to weeds. They stay in maize crops as long as green maize plants are available. After the maize matures, adults feed on pollen resources from a high diversity of weed species. They are strong flyers but most of their flights are short (Coats et al., 1986) and seems to be based on random dispersal within fields of maize (Naranjo, 1994) but a large-scale spread is also possible with sustained flights of a maximum of 24 km on flight mills (Coats et al., 1986). Sustained flights occurred only during the early morning and evening, and short flights occurred during any part of the day (Coats et al., 1986). For the invading European populations, the mean annual dispersal distance varies depending on the authors between 11 and 38 km (and even 60 to 100 km / year for some authors) (Bermond et al., 2013). Under field conditions, mean longevity of adult females varied between 1 month and 1.5 months.
Evening temperatures of about 18°C are optimal for oviposition. Females primarily lay their eggs into the soil of maize fields. The mean lifetime fecundity of D. virgifera virgifera in the field in the USA is approximately 300–400 eggs/female but can strongly vary between years (Toepfer and Kuhlmann, 2005).
Some Eastern United States Corn Belt populations have evolved to lay their eggs in soybean where maize is often grown the following year, despite detrimental effects on beetle survival (Spencer et al., 2021). This behavioural adaptation to annual rotation which allows larval development and adult emergence in the subsequent crop is not known in Europe.
DETECTION AND IDENTIFICATION 2023-04-18
Symptoms
Larval feeding on the roots causes root pruning. As a result, the force needed to pull the plant out of the soil decreases. Plants that are goosenecked (bent at the plant-soil interface) and lodged (tilted in a pronounced manner) are a typical symptom of feeding by D. virgifera. The older larvae burrow in the cortical parenchyma of the roots, and then dig channels in the central vascular tissue. Tunnels in maize roots are thus a characteristic symptom, though they may be due to other species.
Adults feed on leaves as well as on silks, pollen, and immature kernels of maize in the ear tip. On leaves, they remove the epidermis in linear streaks in the same way as Oulema melanopus (the cereal leaf beetle), or chew linear holes into the leaf tissue. On silks, the symptom is called ‘silk clipping’. Severely clipping silks during pollen shed may interfere with maize plant pollination and result in poorly filled ears. No specific symptoms are associated with young kernel consumption.
Morphology
Egg
Eggs are oval and are light yellow when freshly oviposited, turning brown before hatching ; they measure 0.6 mm in length and 0.35 mm in width. It has been noted by Atyeo et al. (1964) that the surface of the egg of rootworm pests is covered with primary polygons and sometimes secondary ridges, useful indicators for identification using the scanning electron microscope. D. virgifera virgifera and D. virgifera zeae are readily distinguished from Diabrotica longicornis by the absence of secondary ridges inside angular polygons for the first two species; the pits inside polygons of D. longicornis are rounded, evenly spaced and there are about 6-12 per polygon. Chorion sculpturing of D. virgifera virgifera is indistinguishable from that of D. virgifera zeae (Krysan et al., 1980).
Larva
Newly hatched larvae are translucent and almost colourless, while mature larvae are creamy white, with the head and end of the abdomen dark brown (Mitchell et al., 2010). Larvae are 2-3 mm long at emergence and have six very small legs, the last instar measures up to 12-19 mm and has no urogomphi (a paired outgrowth of the last body segment). The identification key of Mendoza and Peters (1964) can be used to differentiate mature larvae of D. virgifera from those of D. undecimpunctata howardi and D. longicornis. To our knowledge, it is not possible to morphologically distinguish the larvae of the two subspecies of D. virgifera.
Pupa
The pupa is about 7.5 mm long and 4.5 mm width, white, becoming yellow with age and looking like an adult with rudimentary antennae, legs and wings. The tip of the abdomen bears a pair of stout spines, and smaller spines are found dorsally on the other abdominal segments. There is a sexual dimorphism at the pupal stage, female pupae bearing a pair of distinctive papillae on the venter near the apex of the abdomen, whereas such papillae are lacking for males (Krysan and Miller, 1986). Pupae are found in earthen cells in the soil near plant roots.
Adult
Length 4.6-5.9 mm long. Elytra green, vittate, with one sutural and one humeral vittae (often expanding to cover most of elytra), vittae black, humeral calli black. Head basic colouration from chestnut brown to black with filiform antennae. Pronotum yellow or sulphur yellow, deeply bifoveate. Scutellum black or amber brown. Tarsi yellow or yellow ochre. Protibia bicoloured, yellow, outer edge with piceous (i.e. glossy black or glossy brownish-black) or testaceous line, or almost entirely darkened. D. virgifera virgifera is easily distinguished from the subspecies D. virgifera zeae by the distinct black humeral vittae on elytra. The femora of D. virgifera virgifera are bicoloured with dark, chestnut or piceous, outer edge, allowing distinction from D. longicornis and D. barberi which have femora that are unicolourous green or yellow. A full description of the adult is available in Derunkov et al. (2013).
Detection and inspection methods
The detection of D. virgifera virgifera requires the collection of insect specimens for analysis. Visual examination of imported plant commodities and soil or washing the roots and sifting soil to recover hidden stages (i.e. larvae or pupae) is possible, but the effectiveness of these methods is quite uncertain, especially in cases of very low levels of infestation. Moreover, in case of interception of a larva, there is no morphological method to differentiate the two subspecies of D. virgifera; and while DNA sequences are available in some databases (NCBI or BOLD), they are not supported by validation data. Yellow sticky traps baited with chemical kairomone attractant and cucurbitacin-baited traps can be used for the monitoring of production sites (Jackson et al., 2005; Luna, 2006; Alston and Worwood, 2012). However, the effectiveness of these methods is quite uncertain, especially in cases of very low levels of infestation.
For detection of adults, several trap types are available. Traps baited with a synthetic pheromone that attracts males of D. virgifera virgifera are widely used for ‘early detection’ in new regions and to monitor spread all over Europe (Toth et al., 2010). Occasionally, the pheromone is combined with a floral lure and can therefore attract both males and females to the trap. Visual traps (non-baited, yellow-coloured traps) are less attractive than pheromone traps but appropriate for population estimation (Komaroni et al., 2010). Adults of D. virgifera virgifera can be identified using the EPPO diagnostic protocol PM7/036(2)(EPPO, 2017). Microsatellites have been developed and are sufficiently polymorphic to be used for diagnostics and surveillance performed during pest management programmes (Waits and Stolz, 2008).
In the field, inspection could also consist of searching for the presence of typical damage such as goosenecked and lodged plants (see Symptoms). Any such symptom should be followed by further investigations for the presence of the pest.
PATHWAYS FOR MOVEMENT 2022-12-09
While the larvae move relatively little, the adults of D. virgifera virgifera are good fliers and can travel readily from field to field, or migrate over longer distances, moving with weather features such as cold fronts (Grant and Seevers, 1989). However, their flight potential is not sufficient to spread from North or Central America to the EPPO region. Long distance dispersal of adults is associated with hitchhiking behaviour and airplanes are believed to have been responsible for transatlantic introductions of D. virgifera virgifera in Europe (Miller et al., 2005).
D. virgifera virgifera may also move in international trade as immature stages (eggs, larvae or pupae) in soil and growing media (with or without host plants). But survival of immature stages on roots of host plants, or in soil (either as a commodity on its own, or when accompanying plants for planting in international trade), is not known. Considering that the rearing of Diabrotica species in laboratory facilities requires experience and attentiveness of the operator and also a good knowledge of the ecological needs of the insect to promote the growth and development of the insect (Jackson, 1986), the survival rate is probably very low.
PEST SIGNIFICANCE 2023-04-18
Economic impact
Both adults and larvae attack maize, but the main economic losses are caused by the root damage inflicted by the feeding larvae. In the USA, the cost of soil insecticides to control larval damage to roots, and of aerial sprays to reduce adult damage to silks, when combined with crop losses, can approach 1000 million USD annually (Krysan and Miller, 1986). In that country, there are large disparities in yield losses reported in the literature. They generally range between 10 and 40% or in extreme cases even 90%. Calvin et al. (2001) estimated yield losses for untreated fields in the north-eastern part of the USA to be 6.5 %.
In Europe, Baufeld and Enzian (2005) estimated a potential yield loss worth 147 million EUR for a group of eight countries (Austria, Belgium, France, Germany, Italy, Luxemburg, the Netherlands and Switzerland) for one year assuming a damage level of 10%. However, at present no EU countries are reporting direct economic damage on a large scale. In many countries, solutions based on crop rotation are regularly implemented, avoiding the use of additional insecticides. Therefore, D. virgifera virgifera has not become as serious a pest as was expected when it was discovered in much of Europe (Bazoc et al., 2021).
In the EPPO region, in countries where D. virgifera virgifera is regulated, there will be some economic impact related to enforcement of the regulation: for the monitoring, the management of isolated outbreaks or the cost associated with the containment programmes.
Control
Levine and Oloumi-Sadeghi (1991) refer to the following approaches in their detailed review of integrated pest management (IPM) options for this pest: crop rotation, tillage, choice of planting and harvesting dates, host-plant resistance, biological control, as well as control of adults and larvae with insecticides. Present IPM strategies rely on the monitoring of pest populations, use of economic thresholds and integration of the different control methods. Crop rotation is the recommended management strategy because D. virgifera virgifera females have a strong affinity to oviposit (in autumn) in maize fields and larvae cannot survive (in the following spring) without maize, except on specific grass species.
One strategy practiced by farmers in the USA consists of applying a soil insecticide on a preventive basis (Calvin, 2017). Indeed, this strategy reduces the risk of reduction in maize yields because of corn rootworms, but it may not be the optimal strategy because a lot of fields which are treated do not justify management. In Pennsylvania (USA), surveying sites for adult beetles from the previous summer is recommended to monitor pest population densities. Regional bulletins are provided to the producers to better define the starting date of the survey. The decision to apply treatments against D. virgifera virgifera populations is based on economic thresholds depending on whether maize is produced in that field for the first time since rotation (one beetle per plant on average) or is produced on a continuous basis (1.5 beetle per plant on average). In addition, numbers of D. virgifera virgifera adults caught on yellow sticky traps show a good correlation with the subsequent year’s larval damage in maize fields. Whether economic thresholds will be reached can also be assessed on maize one year in advance by counting the number of larvae per plant in root-soil samples or by rating the root damage based on the standard IOWA 1-6 scale (Hills and Peters, 1971) or any equivalent system. If necessary, chemical control can be performed with liquid and granular soil insecticides applied at planting as a band application over the maize rows and incorporated into the soil, with seed treatments (note that environmental and non-target concerns have led to neonicotinoid bans or restrictions in many countries) or with post-emergence insecticide application (Rozen and Ester, 2010; Meinke et al., 2021).
The application of foliar insecticides to suppress adults of D. virgifera virgifera is usually not necessary. However, the adult management strategy worked well in the western U.S. Corn Belt between the 1960s and the early 1990s: this was used primarily to protect maize during the pollination period from excessive adult silk feeding, and/or to reduce female density and associated oviposition to reduce potential larval injury the following season in the succeeding maize crops. However, reports of adult control failures increased during the 1990s and this management strategy used as a stand-alone measure has now gradually been abandoned.
In 2003, the first genetically modified maize hybrids were commercially released in the USA. These hybrids contain a gene that codes for an insecticidal protein that is toxic to corn rootworm larvae that feed on their roots. Later, several other rootworm-active proteins were registered as single-protein hybrids. The high efficacy and convenience of transgenic maize facilitated widespread adoption by US growers but led to field-evolved resistance to the Cry3Bb1 protein and then to any Cry3 proteins. Field-evolved resistance to Cry34 has been also documented. Transgenic hybrids expressing two Bt proteins (Cry3Bb1 + Cry34/35Ab1) have been utilized by maize growers to mitigate resistance evolution in recent years but Reinders et al., (2022) reported the first confirmed cases of field-evolved resistance to Cry3Bb1 + Cry34/35Ab1 maize in Nebraska.
Due to the restriction of neonicotinoid seed coatings and the high toxicity of tefluthrin-based soil insecticides, biological control solutions have been developed against the larvae of D. virgifera virgifera (Toepfer and Toth, 2020) including entomopathogenic fungi and nematodes. Nematode-based novel biocontrol products (Heterorhabditis bacteriophora) can, in general, control D. virgifera virgifera larvae with comparable efficacies to conventional insecticides in laboratory conditions. Unfortunately, they show inconsistent and fluctuating levels of efficacy at reducing D. virgifera virgifera populations and at preventing root damage when applied under field conditions, which may be due to locally varying abiotic and environmental factors (Toth et al., 2020).
Phytosanitary risk
Maize, the main host of D. virgifera virgifera, is widely cultivated in the EPPO region and is of particular importance in areas such as the Danube basin, the Po valley in Italy or the south-west of France. The potential distribution of D. virgifera virgifera in the EU has been assessed in several studies with many different approaches including correlative and mechanistic models (Aragon et al., 2010; Kriticos et al., 2012; Marchioro and Krechemer, 2018). These studies generally conclude that most of Europe from the Iberian Peninsula to Eastern Russia is predicted as optimal for establishment (Marchioro and Krechemer, 2018). Nearly all maize-growing areas in the EPPO region are at risk, particularly those in the more continental areas of Central Europe, where conditions most resemble those of Mid-Western USA.
D. virgifera virgifera has now been established in Europe for more than 30 years and is a potentially serious threat in several EPPO countries. It has continued to multiply and spread throughout EPPO region to new countries and increasing population densities. However, D. virgifera virgifera has not become as serious a pest as expected when it was discovered on the majority of European territories (Bazoc et al., 2021). It can be effectively managed by different control strategies, based on crop rotation, which is the most effective measure for maintaining populations permanently below the damage threshold. However, vigilance is necessary in countries where maize is an important crop and where beetle populations are still restricted to few regions (such as France and Spain).
PHYTOSANITARY MEASURES 2023-04-18
In countries where D. virgifera virgifera is absent or does not occupy its full ecological niche, phytosanitary measures at import and measures aimed at eradicating or containing pest populations may be applied. This would prevent (further) entry and delay spread (and consequently impact) as much as possible.
Adults actively fly and are unlikely to remain on the plants during harvesting or trade. Entry into the EPPO region at the adult stage with host plants seems therefore unlikely. Nevertheless, the EFSA PLH (2019) has considered that fresh maize cobs and forage/green maize are open pathways that could provide a route of entry for adults of the subspecies D. virgifera zeae. Some control measures could be implemented to mitigate this risk at the place of production (such as chemical treatments), or on the commodity before export (such as treatment of plants by storage in modified atmosphere). Hitchhiking e.g. via airplanes is also possible. In contrast, eggs and larval stages are hidden in soil and thus could travel and remain undetected during import controls. However, larval host plants are limited to few species, the main one being maize which is not traded with soil. Moreover, as is the case in several EPPO countries (e.g. in the EU; EU, 2019), a prohibition on the import of soil or growing media from most third countries should prevent the entry of immature stages. Even though current national regulations in the EPPO region may not cover the true host range of D. virgifera virgifera, phytosanitary measures are widely applied to plants for planting and soil. The possible entry pathways can therefore be considered as already partially closed in several EPPO countries.
When eradication measures are taken, the main components should consist of the definition of a demarcated area (infested area and buffer zone) with restriction in the movement of fresh maize and soil, obligation of crop rotation, requirement to clean the agricultural machinery before it leaves the infested area and removing of volunteer maize plants in succeeding crops. Adults should be monitored in the demarcated areas to enable the rapid detection of new outbreaks and to assess the spread. For successful eradication, it is also important that farmers are aware of the risk, trained about the biology, damage, economics and control options. In addition to this, they should understand the consequence of D. virgifera virgifera in maize agro-ecosystems. The buffer zone should be placed in low intensive maize growing areas (natural barriers), making measures based on interruption of continuous maize growing (together with growing farmer awareness of D. virgifera virgifera) more acceptable and feasible to limit the further spread of the pest.
REFERENCES 2022-12-09
Alston D & Worwood D (2012) Western Striped and Western Spotted Cucumber Beetles. All Current Publications. Paper 987. https://digitalcommons.usu.edu/extension_curall/987 [accessed on 29 September 2022].
Aragón P, Baselga A & Lobo J M (2010) Global estimation of invasion risk zones for the Western corn rootworm Diabrotica virgifera virgifera: integrating distribution models and physiological thresholds to assess climatic favourability. Journal of Applied Ecology 47, 1026–1035.
Atyeo WT, Weekman GT & Lawson DE (1964) The identification of Diabrotica species by chorion sculpturing. Journal of the Kansas Entomological Society 37, 9-11.
Baufeld P & Enzian S (2005) Diabrotica virgifera virgifera, its potential spread and economic and ecological consequences in Germany. In Symposium on the Introduction and the Spread of Invasive Species, Humboldt University, Berlin, Germany. 6pp.
Bažok R, Lemić D, Chiarini F & Furlan L (2021) Western corn rootworm (Diabrotica virgifera virgifera LeConte) in Europe: Current status and sustainable pest management. Insects 12(3), 195.
Bermond G, Blin A, Vercken E, Ravigné V, Rieux A, Mallez S, Morel-Journel T & Guillemaud T (2013) Estimation of the dispersal of a major pest of maize by cline analysis of a temporary contact zone between two invasive outbreaks. Molecular ecology 22(21), 5368-5381.
Branson TF (1976) The selection of a non-diapause strain of Diabrotica virgifera (Coleoptera: Chrysomelidae). Entomologia Experimentalis et Applicata 19(2), 148-154.
Branson TF & Krysan JL (1981) Feeding and oviposition behavior and life cycle strategies of Diabrotica: an evolutionary view with implications for pest management. Environmental Entomology 10(6), 826-831.
Branson TF & Ortman EE (1967) Host range of larvae of the Western corn rootworm. Journal of Economic Entomology 60(1), 201-203.
Branson TF & Ortman EE (1970) The host range of larvae of the Western corn rootworm: further studies. Journal of Economic Entomology 63(3), 800-803.
Calvin DD (2017) Western and Northern Corn Rootworm Management in Pennsylvania. PennState Extension. https://extension.psu.edu/western-and-northern-corn-rootworm-management-in-pennsylvania [accessed on 30 September 2022].
Calvin DD, Roth GW, Hyde J, Kuldau G & Voight D (2001) Incorporating Bt-corn Hybrids into IPM Programs for Field Crop Farmers in the Northeastern United States. https://portal.nifa.usda.gov/web/crisprojectpages/0188864-incorporating-bt-corn-hybrids-into-field-crop-ipm-progams.html [accessed on 30 September 2022].
Chiang HC (1973) Bionomics of the Northern and Western corn rootworms. Annual Review of Entomology 18, 47-72.
Ciosi M, Miller N J, Kim K S, Giordano R, Estoup A & Guillemaud T (2008) Invasion of Europe by the Western corn rootworm, Diabrotica virgifera virgifera: multiple transatlantic introductions with various reductions of genetic diversity. Molecular Ecology 17(16), 3614-3627.
Coats SA, Tollefson JJ & Mutchmor JA (1986) Study of migratory flight in the Western corn rootworm (Coleoptera: Chrysomelidae). Environmental Entomology 15(3), 620-625.
Derunkov A, Konstantinov A, Tishechkin A, Hartje L & Redford AJ (2013) Diabrotica ID: Identification of Diabrotica species (Coleoptera: Chrysomelidae) from North and Central America. USDA Animal and Plant Health Inspection Service, Plant Protection and Quarantine Center for Plant Health Science and Technology, USDA Agricultural Research Service, University of Maryland, and Louisiana State University. Available at http://idtools.org/id/beetles/diabrotica/ [accessed on 29 September 2022].
EFSA PLH (2019) Panel on Plant Health (PLH), Bragard C, Dehnen-Schmutz K, Di Serio F, Gonthier P, Jacques M-A, Jaques Miret JA, Justesen AF, Magnusson CS, Milonas P, Navas-Cortes JA, Parnell S, Potting R, Reignault PL, Thulke H-H, Van der Werf W, Civera AV, Yuen J, Zappala L, Kertesz V, Maiorano A, Streiss lF & MacLeod A (2019) Scientific Opinion on the pest categorisation of Diabrotica virgifera zeae. EFSA Journal 17(11),5858, 29 pp. https://doi.org/10.2903/j.efsa.2019.5858
Ellsbury MM, Banken KR, Clay SA & Forcella F (2005) Interactions among Western corn rootworm (Coleoptera: Chrysomelidae), yellow foxtail, and corn. Environmental Entomology 34 (3), 627-634.
EPPO (2017) EPPO Standard PM 7/36 (2) Diabrotica virgifera virgifera. Diagnostics. EPPO Bulletin 47, 164-173.
EPPO (2022) Diabrotica virgifera zeae. EPPO datasheets on pests recommended for regulation. Available online. https://gd.eppo.int
EU (2019) Commission implementing Regulation (EU) 2019/2072 establishing uniform conditions for the implementation of Regulation (EU) 2016/2031 of the European Parliament and the Council, as regards protective measures against pests of plants, and repealing Commission Regulation (EC) No 690/2008 and amending Commission Implementing Regulation (EU) 2018/201. Official Journal of the European Union L319 (2019).
Fora CG & Lauer KF (2013) Host plants for the Western corn rootworm Diabrotica virgifera virgifera (Coleoptera: Chrysomelidae). Romanian Agricultural Research 30, 291-295.
Gillette CP (1912) Diabrotica virgifera Lec. as a corn root-worm. Journal of Economic Entomology 5(2), 364-366.
Grant RH & Seevers KP (1989) Local and long-range movement of adult Western corn rootworm (Coleoptera: Chrysomelidae) as evidenced by washup along southern Lake Michigan shores. Environmental Entomology 18(2), 266-272.
Gray ME, Sappington TW, Miller NJ, Moeser J & Bohn MO (2009) Adaptation and invasiveness of Western corn rootworm: intensifying research on a worsening pest. Annual Review of Entomology 54, 303-321.
Guillemaud T (2007) Diabr-Act - Harmonise the strategies for fighting Diabrotica virgifera virgifera. Diabr-Act – Deliverable D01.09. Literature survey on WCR ecology finalised (WP1 Task 2.1) EU Funded Project FP6-2004-SSP-4-022623. 92pp.
Hills TM & Peters DC (1971) A Method of Evaluating Postplanting Insecticide Treatments for Control of Western Corn Rootworm Larvae. Journal of Economic Entomology 64(3), 764–765.
Horn GH (1893) The Galerucini of boreal America. Transactions of the American Entomological Society 20, 57-144.
Jackson DM, Sorensen KA, Sorenson CE & Story RN (2005) Monitoring cucumber beetles in sweet potato and cucurbits with kairomone-baited traps. Journal of Economic Entomology 98, 159-170.
Jackson JJ (1986) Rearing and handling of Diabrotica virgifera and Diabrotica undecimpunctata howardi. In Methods for the study of pest Diabrotica (eds Krysan), pp. 25–37, Springer, New York (US).
Jackson JJ & Elliott NC (1988) Temperature-dependent development of immature stages of the Western corn rootworm, Diabrotica virgifera virgifera (Coleoptera: Chrysomelidae). Environmental Entomology 17(2), 166-171.
Jaksons R, Falkner K & Moltchanova E (2022) Modelling the emergence dynamics of the Western corn rootworm beetle (Diabrotica virgifera virgifera). Scientific reports 12(1), 1-8.
Komáromi JP, Kiss J, Pálinkás Z &Toepfer S (2010) Western corn rootworm in Europe: integrated pest management is the only sustainable solution.EU grant (Project number: 031499), ENDURE Maize Case Study – Guide Number 2. 8pp.
Kriticos DJ, Reynaud P, Baker RHA & Eyre D (2012) Estimating the global area of potential establishment for the Western corn rootworm (Diabrotica virgifera virgifera) under rain‐fed and irrigated agriculture. EPPO Bulletin 42(1), 56-64.
Krysan JL (1978) Diapause, quiescence, and moisture in the egg of the Western corn rootworm, Diabrotica virgifera. Journal of Insect Physiology 24, 535-540.
Krysan JL, Branson TF & Diaz Castro G (1977) Diapause in Diabrotica virgifera (Coleoptera: Chrysomelidae): a comparison of eggs from temperate and subtropical climates. Entomologia Experimentalis et Applicata 22, 81-89.
Krysan JL, Smith RF, Branson TF & Guss PL (1980) A new subspecies of Diabrotica virgifera: description, distribution and sexual compatibility. Annals of the Entomological Society of America 73, 123-130.
Krysan, JL & Miller TA (1986) Methods for the study of pest Diabrotica. Springer-Verlag, New York, USA, 259pp.
Levine E & Oloumi-Sadeghi H (1991) Management of diabroticite rootworms in corn. Annual Review of Entomology 36, 229-255.
Levine E, Oloumi-Sadeghi H& Ellis CR (1992) Thermal requirements, hatching patterns, and prolonged diapause in Western corn rootworm (Coleoptera: Chrysomelidae) eggs. Journal of Economic Entomology 85(6), 2425-2432.
Lombaert E, Ciosi M, Miller NJ, Sappington TW, Blin A & Guillemaud T (2018) Colonization history of the Western corn rootworm (Diabrotica virgifera virgifera) in North America: insights from random forest ABC using microsatellite data. Biological invasions 20(3), 665-677.
Ludwig KA & Hill RE (1975) Comparison of gut contents of adult Western and Northern corn rootworms in Northeast Nebraska. Environmental Entomology 4(3), 435-438.
Luna J (2006) Development of an IPM Program for Western Spotted Cucumber Beetle in Vegetable Cropping Systems. Report to the Oregon Processed Vegetable Growers and the Agricultural Research Foundation. Available at: https://ir.library.oregonstate.edu/downloads/tt44pn501 [accessed on 29 September 2022].
Marchioro CA & Krechemer FS (2018) Potential global distribution of Diabrotica species and the risks for agricultural production. Pest management science 74 (9), 2100-2109.
Mazzoni E, Alma A, Cravedi P, Conti M & Michelatti G (2007) Il caso della diabrotica del mais. Il caso della diabrotica del mais, Supplemento a «I Georgofili. Atti dell’Accademia dei Georgofili» - Serie VIII - Vol. 4, 105-122.
Meinke LJ, Souza D & Siegfried BD (2021) The use of insecticides to manage the Western corn rootworm, Diabrotica virgifera virgifera, LeConte: history, field-evolved resistance, and associated mechanisms. Insects 12(2), 112.
Mendoza CE & Peters DC (1964) Species differentiation among mature larvae of Diabrotica undecimpunctata howardi, D. virgifera and D. longicornis. Journal of the Kansas Entomological Society 37, 123-125.
Miller N, Estoup A, Toepfer S, Bourguet D, Lapchin L, Derridj S, Kim KS, Reynaud P, Furlan L & Guillemaud T (2005) Multiple transatlantic introductions of the Western corn rootworm. Science 310, 992.
Mitchell SB, Ciha AJ & Tollefson JJ (2010) Corn Rootworm Biology and Management. Unpublished work. Available at: https://masters.agron.iastate.edu/files/mitchellsteven-cc.pdf [accessed on 29 September 2022].
Moeser J (2003) Nutritional ecology of the invasive maize pest Diabrotica virgifera virgifera LeConte in Europe (Doctoral Dissertation zur Erlangung des Doktorgrades der Fakultät für Agrarwissenschaften der Georg-August Universität Göttingen. 90p.
Naranjo SE (1994) Flight orientation of Diabrotica virgifera virgifera and D. barberi (Coleoptera: Chrysomelidae) at habitat interfaces. Annals of the Entomological Society of America 87 (3), 383-394.
Reinders JD, Reinders EE, Robinson EA, French BW & Meinke LJ (2022) Evidence of Western corn rootworm (Diabrotica virgifera virgifera LeConte) field‐evolved resistance to Cry3Bb1+ Cry34/35Ab1 maize in Nebraska. Pest Management Science 78(4), 1356-1366.
Schaafsma AW, Whitfield GH & Ellis CR (1991) A temperature-dependent model of egg development of the Western corn rootworm, Diabrotica virgifera virgifera LeConte (Coleoptera, Chrysomelidae). The Canadian Entomologist 123(6), 1183-1197.
Spencer JL, Mabry TR, Levine E & Isard SA (2021) Soybean foliage consumption reduces adult Western corn rootworm (Diabrotica virgifera virgifera)(Coleoptera: Chrysomelidae) survival and stimulates flight. Journal of Economic Entomology 114, 2390–2399.
Szalai M, Papp Komáromi J, Bažok R, IgrcBarčić J, Kiss J & Toepfer S (2010) Generational growth rate estimates of Diabrotica virgifera virgifera populations (Coleoptera: Chrysomelidae). Journal of Pest Science 84(1), 133-142.
Toepfer S & Kuhlmann U (2005) Natural mortality factors acting on Western corn rootworm populations: A comparison between the United States and Central Europe. In Western Corn Rootworm: Ecology and Management (eds Vidal, S. et al.), 95–119
Toepfer S & Kuhlmann U (2006) Constructing life‐tables for the invasive maize pest Diabrotica virgifera virgifera (Col.; Chrysomelidae) in Europe. Journal of Applied Entomology 130(4), 193-205.
Toepfer S & Toth S (2020) Entomopathogenic nematode application against root-damaging Diabrotica larvae in maize: What, when, and how. Microbial and Nematode Control of Invertebrate Pests. IOBC-WPRS Bulletin 150, 185-188.
Toth M, Ujvary I & Imrei Z (2010) 8‐Methyldecan‐2‐yl acetate inhibits response to the pheromone in the Western corn rootworm Diabrotica v. virgifera. Journal of Applied Entomology 134(5), 462-466.
Toth S, Szalai M, Kiss J & Toepfer S (2020) Missing temporal effects of soil insecticides and entomopathogenic nematodes in reducing the maize pest Diabrotica virgifera virgifera. Journal of Pest Science 93(2), 767-781.
Van Rozen K & Ester A (2010) Chemical control of Diabrotica virgifera virgifera LeConte. Journal of Applied Entomology 134(5), 376-384.
Waits ER & Stolz U (2008) Polymorphic microsatellite loci from Northern and Mexican corn rootworms (Insecta: Coleoptera: Chrysomelidae) and cross‐amplification with other Diabrotica spp. Molecular ecology resources 8, 707-709.
Wilson TA & Hibbard BE (2004) Host suitability of non-maize agroecosystem grasses for the Western corn rootworm (Coleoptera: Chrysomelidae). Environmental Entomology 33(4), 1102-1108.
ACKNOWLEDGEMENTS 2023-04-18
This datasheet was extensively revised in 2023 by Philippe Reynaud. His valuable contribution is gratefully acknowledged.
How to cite this datasheet?
Datasheet history 2023-04-18
This datasheet was first published in 1997 in the second edition of 'Quarantine Pests for Europe' (as Diabrotica virgifera) and revised in 2023. It is now maintained in an electronic format in the EPPO Global Database. The sections on 'Identity', ‘Hosts’, and 'Geographical distribution' are automatically updated from the database. For other sections, the date of last revision is indicated on the right.
CABI/EPPO (1997) Quarantine Pests for Europe (2nd edition). CABI, Wallingford (GB).
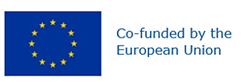