Diabrotica barberi(DIABLO)
EPPO Datasheet: Diabrotica barberi
IDENTITY
Authority: Smith & Lawrence
Taxonomic position: Animalia: Arthropoda: Hexapoda: Insecta: Coleoptera: Chrysomelidae
Other scientific names: Diabrotica longicornis barberi Smith & Lawrence
Common names in English: northern corn rootworm
view more common names online...
Notes on taxonomy and nomenclature
The subfamily Galerucinae (Coleoptera; Chrysomelidae) includes the economically important genus Diabrotica which comprises over 400 described species (including 107 valid species in North and Central America), and is one of the most speciose leaf beetle genera in the New World. Many Diabrotica species feed on flowers, leaves and roots of a wide variety of herbaceous plants, including agricultural crops, vegetables, fruits and ornamentals and are vectors of plant diseases (Derunkov et al., 2013) such as Squash mosaic virus (Krysan & Miller, 1986) or Fusarium spp. (Chiang, 1973). A key to separate Diabrotica from related genera is presented by Derunkov et al. (2015) but despite their bright colours and various patterns, Diabrotica species are notoriously difficult to identify at the species level.
Diabrotica barberi Smith & Lawrence (the northern corn rootworm) was originally described as Galleruca longicornis by Say in 1824 and was subsequently transferred to the genus Diabrotica Chevrolat, 1843, considered only as a subspecies of Diabrotica longicornis (Say). However, based on laboratory and field studies, Krysan et al. (1983) elevated the taxon to species level. They concluded that even when the two species occur in the same geographic areas, adults are found in different habitats and they show a clear sexual isolation that supports the decision to elevate D. barberi to the species level.
EU Categorization: A1 Quarantine pest (Annex II A)
view more categorizations online...
EPPO Code: DIABLO
HOSTS 2020-11-25
Northern corn rootworm larvae have a rather restricted host range (stenophagous) and develop only on plants in the family Gramineae. Corn (Zea mays) is the only crop regularly attacked by the larvae, which feed on roots. However, development can also occur to a lesser extent on millet (Panicum miliaceum), rice (Oryza sativa) and spelt (Triticum spelta). It is worth noting that the historical range of northern corn rootworm likely predates the introduction of maize into the region by Native Americans and colonizing farmers of European origin. Northern corn rootworm larvae are thought to have primarily used unknown host plants before this time. Currently there is no information on the original host(s) of the northern corn rootworm, other than that it can develop on a number of grass species (Oyediran et al., 2008). D. barberi larvae fed on and developed on most of the prairie grass species evaluated by Oyediran et al. (2008) under glasshouse conditions but adult recovery was extremely low.
Adults show a comparable range of host preference, with corn kernels, corn silk and corn tassel tissue favouring survival and reproduction, but maize leaves are not the preferred food of adults (Ludwig and Hill, 1975). Blossoms from Solidago canadensis, Cucurbita spp. and Helianthus annuus are also suitable. Additionally, adults feed on pollen from plants in the families Gramineae, Compositae, Leguminosae, and Cucurbitaceae.
Host list: Agropyron cristatum, Bouteloua curtipendula, Bouteloua dactyloides, Elymus canadensis, Elymus smithii, Elymus trachycaulus, Eragrostis curvula, Hordeum vulgare, Oryza sativa, Panicum miliaceum, Setaria pumila, Setaria viridis, Sorghastrum nutans, Spartina pectinata, Thinopyrum elongatum, Thinopyrum intermedium subsp. intermedium, Thinopyrum intermedium, Triticum aestivum, Triticum spelta, Zea maysGEOGRAPHICAL DISTRIBUTION 2020-11-25
D. barberi is present and native to the Nearctic region only and has never been introduced into another area. It was first discovered attacking maize in Colorado and has since spread Midwest, Northeast and Southeast.
BIOLOGY 2020-11-25
Diabrotica barberi displays a single generation per year, with the egg stage overwintering but a portion of eggs within the population show an extended diapause trait that causes eggs to overwinter for 2 years and occasionally even for 3–4 years (French et al., 2014).
Eggs are laid in sets of 25–31 eggs by the female and hatch in late spring and early summer. Larvae feed on the roots of maize where they have three instars (Hammack et al., 2003). Development from egg to adult can be completed in laboratory studies in the range 15–31.5°C and the lower developmental threshold is estimated to be 10.2°C with 865 accumulated degree-days (base 10.2°C) needed from hatching to adult appearance in the field (Woodson and Jackson, 1996). Upon emergence from the soil, adults feed on maize tassels, silks and ear tips. Adult populations peak in the fields while maize is flowering (Lance et al., 1989). As the floral structures of maize dry, females abandon maize fields (Lance et al., 1989) and become increasingly abundant on the flowers of weeds, prairie forbs and crops other than maize listed above. According to Naranjo and Sawyer (1987), mean female and male longevity were similar, ranging from approximately 90 days at 17.5°C to 42 days at 30°C.
DETECTION AND IDENTIFICATION 2020-11-25
Symptoms
D. barberi larvae feed on the roots of corn, which can inhibit the plant’s capacity to uptake water and nutrients from the soil, lead to plant instability (lodging), and cause yield loss (Geisert and Meinke, 2013).Tunnels in maize roots are thus a characteristic symptom, though they may be due to other species. High levels of infestation by adults can cause additional yield losses by damaging corn silks, which interferes with pollination, and by feeding on developing kernels. In corn-growing areas in the late season when corn silks and kernels are dry, beetles are also seen on a great variety of flowering plants close to the corn fields where they feed on pollen (Lance et al., 1989) but the occurrence of specific symptoms on these other plants is not reported in the literature.
Morphology
Egg
The whitish eggs have a typical ovoid shape and measure approximately 0.6 mm long and 0.4 mm wide. They are covered with a network of polygons (Capinera, 2008), with each polygon containing small pits. The pattern and sculpturing of the surface are useful taxonomic characters for identification and description (Eben and Barbercheck, 1997). Descriptions have been published for several species of Diabrotica, including D. virgifera and D. barberi (see Krysan & Miller, 1986 for scanning electron micrographs of portions of eggshell).
Larva
The larva is elongate and cylindrical, tapering toward the head. The body is white, bearing relatively few hairs or spines. The head capsule, thoracic and anal plates are yellowish brown. The three pairs of legs are brownish and terminate in a single claw. The posterior end of the body bears a single retractile extension or tubercle (Capinera, 2008). Inability to morphologically distinguish Northern from western corn rootworm (Diabrotica virgifera virgifera) during early larval stages is highlighted by Hammack et al. (2003). Nevertheless, Becker and Meinke (2008) differentiate western corn rootworm and northern corn rootworm larvae (at all stages) using a specific measurement on the sclerotized head capsule of each species with a diagnostic accuracy between 97.5 % and 100.0 % depending on the stage.
Adult
Body length 4.8-5.6 mm. Head basic colour yellow. Antennae filiform, pronotum green or yellow, quadrate and deeply bifoveate. Scutellum yellow or amber yellow. Elytra green, sometimes humeri and basal third of suture tinged with amber, with five distinct sinuate sulci, strongest behind the humeral callus and extending beyond the middle. Elytral epipleura green, sutural angle of elytra round, punctation scattered, fine. Abdomen yellow, pale olivine or green. Tarsi black, amber brown or chestnut. Tibiae bicolored yellow, outer edge with piceous or testaceous line, or extensively darkened. Femora uniform yellow or olive ocher.
Most adult specimens of D. longicornis, D. barberi, and D. virgifera can be distinguished from one another by the following features: in D. barberi the head, tibia and tarsi are paler than in D. longicornis; femora unicolorous green or flavous in D. barberi, while femora of D. virgifera as a rule bicolored, with outer edges dark, chestnut or piceous; distance from apex to ventral flange of aedeagus in D. v. virgifera is 1.5 - 2.0 times that of D. barberi. A dissection of male genitalia with observation of the shapes of the internal sac sclerites (especially sclerite 4B) differentiates all three species very well (Derunkov et al., 2013) and confirms the morphological identification.
Detection and inspection methods
The detection generally requires the collection of insect specimens for analysis. Inspections for adults during phytosanitary controls in ports and airports and also during field surveys can be performed with unbaited or baited yellow sticky traps (see control section), or failing that, by visual inspection. Eggs and larvae occur in soil and are therefore more difficult to observe than adults in the absence of evocative plant symptoms. Visual examination of soil for insects or washing the roots and sifting soil to recover larvae is possible, but the effectiveness of these methods is quite uncertain, especially in cases of very low levels of infestation.
Adults of D. barberi can be identified using the diagnostic protocol for Diabrotica virgifera virgifera which includes a key to the adults of 13 Diabrotica species which occur in US agriculture (EPPO, 2017). Electrophoretic and molecular techniques are available to distinguish all larval stages of D. barberi (EPPO, 2017) and a method to differentiate western corn rootworm and northern corn rootworm larvae using morphological characters is also reported by Becker and Meinke (2008).
PATHWAYS FOR MOVEMENT 2020-11-25
There are no major means of intercontinental dispersal by trade, since the insects would not normally be expected to be carried by consignments of seeds or grain. This is perhaps the reason why Diabrotica spp. did not appear earlier in Europe or cause concern there. The EFSA Panel on Plant Health performed a pest categorisation of Diabrotica barberi including a full investigation into potential pathways (EFSA, 2019). They concluded that adults could be carried on consignments of fresh sweetcorn (corn cobs) and forage/green maize, for which pathways are not regulated in several EPPO countries (e.g. in the EU). Unfortunately, statistical data on imports are scarce and partial even if imports from the United States and Canada are reported in EUROSTAT in some years. Other plants on which adults feed on pollen could provide potential pathways if transported when in flower. However, pollen hosts are generally wild plants and weeds and are not considered to provide a realistic pathway. The general prohibition on the import of soil for most EPPO countries would prevent the entry of D. barberi larvae in the region. Europhyt records of pest interceptions from 1994 to 4 June 2019 do not report any interceptions of D. barberi in the EU (EFSA, 2019).
In case of introduction of beetles, larval stages would have a low capacity for natural spread. The adult flight behaviour would be the main source of natural dispersal. In the laboratory, the vast majority of flights last less than 1 min for both sexes. Flights over 9-10 min of D. barberi are extremely rare and there is no evidence of long-distance migration (Naranjo, 1990). In contrast to D. virgifera virgifera, D. barberi shows comparatively short but more frequent flights which appear to be closely related to the cyclic nature of their movement between corn and non-corn habitats to secure other sources of pollen.
PEST SIGNIFICANCE 2024-10-29
Economic impact
There is no detailed assessment of the economic impact of Diabrotica barberi in the USA. The annual impact of the three most relevant maize rootworm species in the USA, D. v. virgifera, D. barberi and D. undecimpunctata howardi Barber was estimated to be about 1.17 billion USD in terms of costs of control and yield loss to American maize producers (Sappington et al., 2006) and management costs along with yield losses to growers were estimated to be up to 2 billion USD in 2010 by Wechsler and Smith (2018).
If D. barberi were to be introduced in the EPPO region, impacts could also be expected as a result of the infection and spread of Squash mosaic virus (SqMV, Secoviridae) or Maize chlorotic mottle virus (MCMV) (Butter, 2018) which it vectors. SqMV is already present in Greece, Italy, Morocco, and the Netherlands (EPPO Global Database, 2020).
Control
The control of Diabrotica spp. in maize in North America is a major problem for this crop. Conventional insecticides have been used for management since the 1940s, and can either be applied to the soil at planting to reduce larval feeding on roots or aerially to both decrease silk feeding and suppress adult populations and oviposition (Levine & Oloumi-Sadeghi, 1991). More recently, systemic seed treatments or transgenic maize hybrids that express Bacillus thuringiensis (Bt)-derived insecticidal proteins have been successfully implemented. But rapid adoption of Bt crops has led to concerns over the development of resistant populations and now field-evolved resistance is reported in some North American populations (Calles-Torrez et al., 2019).
The most important cropping practice to manage D. barberi is crop rotation, which normally results in destruction of rootworm populations due to the inability of larvae to survive on crops other than corn. However, some populations of northern corn rootworm have adapted to crop rotation through prolonged egg diapauses, in which eggs remain in diapause and hatch in the spring after two or more winters, potentially reducing the effectiveness of this practice. This behaviour has increased in frequency and geographic distribution since the first report in Illinois in 1932 but remain strongly related to geographic location (French et al., 2014) and crop rotation is still a preferred management practice.
Compared to Diabrotica virgifera, limited research has been done on biological control methods to manage D. barberi, likely due to a shortfall in available eggs for studies (Ludwick and Hibbard, 2016).
Monitoring corn rootworms in cornfields is crucial to help make informed IPM decisions and many different types of traps are available to follow population levels (Calles-Torrez, 2018) with varying performance depending on the trap. Unbaited yellow sticky traps are widely used, and their attractiveness can be enhanced by use of some semiochemicals (Capinera, 2001). The results from various studies suggest that eugenol-trap based sampling might be useful for monitoring and managing D. barberi in a diverse set of habitats (including corn and other plants on which adults feed) (Helser, 2016).
Phytosanitary risk
D. barberi occurs across a range of climatic zones across mid and eastern North America and in case of introduction in the EPPO region (e.g. the EU), could find climates closely related to those in its native range (EFSA, 2019). The EFSA Panel on Plant Health assume that climatic conditions in the EU will not limit the ability of D. barberi to establish. Once introduced, it will easily find maize fields, because Z. mays occurs in large parts of the EPPO region and then spread through short migrations to feed on other plant species when food within the corn fields became scarce. Impact could be expected in terms of costs of control and yield losses to maize producers. However, the final impact could be mitigated by the fact that as D. virgifera is already introduced in the EPPO region, management measures for the two species would overlap.
PHYTOSANITARY MEASURES 2020-11-25
A number of EPPO countries already prohibit the import of soil and growing media from North-America and require that Z. mays plants (including living parts) should be accompanied by a phytosanitary certificate to enter into their territory, indicating that these plants have been inspected and are free from quarantine pests prior to entry (EU, 2019). Requirements for the production of e.g. maize combs or green maize could be based on the production in pest-free zones (pest free areas or place/site of production with a buffer zone) (EFSA, 2019). In case of entry on such plants, the following additional measures could be ordered: chemical treatments of consignments, processing under specified conditions or storage in a modified atmosphere.
Establishment or spread could be prevented or slowed down by crop rotation, soil/seed-applied systemic insecticides to control rootworm larvae, use of maize hybrids containing genes from the soil bacterium Bacillus thuringiensis.
REFERENCES 2020-11-25
Becker SC, & Meinke LJ (2008) A technique to morphologically differentiate larvae of Diabrotica virgifera virgifera and D. barberi (Coleoptera: Chrysomelidae). Journal of the Kansas Entomological Society 81, 77-79.
Butter NS (2018) Insect Vectors and Plant Pathogens. CRC Press. 470 pp.
Calles-Torrez V (2018) Geographic Distribution and Effects of Transgenic Corn Hybrids and Chemical Insecticides on Northern and Western Corn Rootworms (Coleoptera: Chrysomelidae) in North Dakota. [Doctoral dissertation, North Dakota State University]. Institutional Repository at the North Dakota State University. Available at https://library.ndsu.edu/ir/handle/10365/29965
Calles-Torrez V, Knodel J, Boetel MA, French BW, Fuller BW & Ransom, JK (2019) Field-evolved resistance of northern and western corn rootworm (Coleoptera: Chrysomelidae) populations to corn hybrids expressing single and pyramided Cry3Bb1 and Cry34/35Ab1 Bt proteins in North Dakota. Journal of Economic Entomology 112,
Capinera JL (2001) Handbook of Vegetable Pests. Academic Press, San Diego, 729 pp.
Capinera JL (2008) Encyclopedia of entomology, 2nd ed., Vol. 1-4, Springer Science and Business Media, New York: 4346 pp.
Chiang HC (1973) Bionomics of the northern and western corn rootworms. Annual Review of Entomology 18, 47–72.
Derunkov A, Konstantinov A, Tishechkin A, Hartje L & Redford AJ (2013) Diabrotica ID: Identification of Diabrotica species (Coleoptera: Chrysomelidae) from North and Central America. USDA APHIS PPQ Center for Plant Health Science and Technology, USDA Agricultural Research Service, University of Maryland, and Louisiana State University. Available at http://idtools.org/id/beetles/diabrotica/ [28-08-2020]
Derunkov A, Prado LR, Tishechkin A, & Konstantinov AS (2015) New species of Diabrotica Chevrolat (Coleoptera: Chrysomelidae: Galerucinae) and a key to Diabrotica and related genera: results of a synopsis of North and Central American Diabrotica species. Journal of Insect Biodiversity 3, 1–55.
Eben A & Barbercheck ME (1997) Sculpturing of the eggshell of some Mexican Galerucinae (Coleoptera: Chrysomelidae). The Coleopterists' Bulletin 51, 80-85.
EPPO (2017), EPPO Standard PM 7/36 (2) Diabrotica virgifera virgifera. Diagnostics. EPPO Bulletin 47, 164-173.
EPPO Global Database (2020) Distribution map for Squash mosaic virus. Available at https://gd.eppo.int/taxon/SQMV00/distribution [accessed 2020-09-14]
EU (2019) Commission implementing Regulation (EU)
EFSA (2019) Bragard C, Dehnen‐Schmutz K, Di Serio F, Gonthier P, Jacques M‐A, Miret JA, Justesen AF, Magnusson CS, Milonas P, Navas‐Cortes JA, Parnell S, Potting R, Reignault PL, Thulke H‐H, Van der Werf W, Vicent Civera A, Yuen J, Zappalà L, Czwienczek E, MacLeod A. Scientific Opinion on the pest categorisation of Diabrotica barberi. EFSA Journal 17, 5857, 28 pp. Available at https://doi.org/10.2903/j.efsa.2019.5857
French BW, Coates BS, Sappington TW (2014) Inheritance of an extended diapause trait in the Northern corn rootworm, Diabrotica barberi (Coleoptera: Chrysomelidae). Journal of Applied Entomology 138, 213-221.
Geisert RW & Meinke LJ (2013) Frequency and distribution of extended diapause in Nebraska populations of Diabrotica barberi (Coleoptera: Chrysomelidae). Journal of economic entomology 106,
Hammack L, Ellsbury MM, Roehrdanz RL & Pikul Jr JL (2003) Larval sampling and instar determination in field populations of northern and western corn rootworm (Coleoptera: Chrysomelidae). Journal of economic entomology 96,
Krysan JL, Smith RF, Guss PL (1983) Diabrotica barberi (Coleoptera: Chrysomelidae) elevated to species rank based on behavior, habitat choice, morphometrics, and geographical variation of color. Annals of the Entomological Society of America 76, 197-204.
Krysan JL & Miller TA (1986) Methods for the study of pest Diabrotica. Springer-Verlag, New York, USA.
Lance DR, Elliott NC & Hein GL (1989) Flight activity of Diabrotica spp. at the borders of cornfields and its relation to ovarian stage in D. barberi. Entomologia experimentalis et applicata, 50, 61-67.
Levine E & Oloumi-Sadeghi H (1991) Management of diabroticite rootworms in corn. Annual Review of Entomology 36, 229-255.
Ludwick DC & Hibbard BE (2016) Rootworm management: status of GM traits, insecticides and potential new tools. CAB Reviews 11, 1-10.
Ludwig KA & Hill RE (1975) Comparison of Gut Contents of Adult Western and Northern Cora Rootworms in Northeast Nebraska. Environmental Entomology 4, 435-438.
Naranjo SE (1990) Comparative flight behavior of Diabrotica virgifera virgifera and Diabrotica barberi in the laboratory. Entomologia Experimentalis et Applicata 55, 79-90.
Naranjo SE & Sawyer AJ (1987) Reproductive biology and survival of Diabrotica barberi (Coleoptera: Chrysomelidae): effect of temperature, food, and seasonal time of emergence. Annals of the Entomological Society of America 80, 841-848.
Oyediran IO, French BW, Clark TL, Dashiell KE & Hibbard BE (2008) Prairie grasses as hosts of the northern corn rootworm (Coleoptera: Chrysomelidae). Environmental entomology 37, 247-254.
Sappington TW, Siegfried BD & Guillemaud T (2006) Coordinated Diabrotica genetics research: accelerating progress on an urgent insect pest problem. American Entomologist 52, 90–97.
Wechsler S &, Smith D (2018) Has resistance taken root in US corn fields? Demand for insect control. American Journal of Agricultural Economics 100,
Woodson WD & Jackson JJ (1996) Developmental rate as a function of temperature in northern corn rootworm (Coleoptera: Chrysomelidae). Annals of the Entomological Society of America 89, 226-230.
ACKNOWLEDGEMENTS 2020-11-25
This datasheet was extensively revised in 2020 by Philippe Reynaud. His valuable contribution is gratefully acknowledged.
How to cite this datasheet?
Datasheet history 2020-11-25
This datasheet was first published in 1997 in the second edition of 'Quarantine Pests for Europe' (together with Diabrotica virgifera) and revised in 2020. It is now maintained in an electronic format in the EPPO Global Database. The sections on 'Identity', ‘Hosts’, and 'Geographical distribution' are automatically updated from the database. For other sections, the date of last revision is indicated on the right.
CABI/EPPO (1997) Quarantine Pests for Europe (2nd edition). CABI, Wallingford (GB).
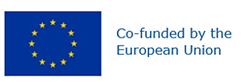